Introduction
Materials and Methods
Chemicals and seed materials
Cultivation of lotus sprouts
Measurement of growth parameters and preparation of sprout powder samples
Color measurement
Determination of total polyphenol content
Determination of Free amino acid content
Determination of DPPH radical scavenging activity
Determination of ABTS radical scavenging activity
Determination of superoxide dismutase (SOD)-like activity
Statistical analysis
Results
Germination rate and sprout length
Color
Free amino acid composition
Antioxidant potential
Discussion
Introduction
Lotus (Nelumbo nucifera Gaertn.), an economically important aquatic herb, is grown in many countries, including Korea. The herb is also recognized as a popular ornamental plant because of its beautiful flowers with appealing fragrance. The seeds, rhizomes, leaves, flowers, stalks, thalamus, and embryos of this plant are utilized as food, tea, natural pigments, and/or healthcare product (Mukherjee et al., 2009; Zhu et al., 2016). The peeled seeds can be eaten fresh or roasted. The powdered seed is used to prepare breads. In addition, different parts of the plants are also used in traditional herbal medicine against different diseases (Onishi et al., 1984). The embryos of lotus seeds are considered to be effective in removing heat from the heart, anchoring the mind, improving spermatorrhea and controlling bleeding (Yu et al., 2013). Some alkaloids found in the embryos possess various biological functions, such as anti-tumor (Poornima et al., 2014; Zhang et al., 2012), anti-inflammatory (Lin et al., 2006), anti-oxidation (Jung et al., 2010; Wu et al., 2011), and sedation (Nishimura et al., 2012; Sugimoto et al., 2008). A number of studies on lotus seeds and other plant parts have been carried out to investigate their nutritional and phytochemical potentials. However, studies on the effect of different methods of sprouting on the nutritional and antioxidant potential of lotus seeds are lacking.
Sprouting is a simple technique and can be used to produce seed sprouts without the use of any expensive instruments. Sprouting usually enhances the nutritional value (Gulewicz et al., 2008) and reduces anti-nutritional factors of seeds, making the sprouts more appropriate for human health (Luo et al., 2013). The protein/amino acid digestibility is increased but the anti-nutritional factors are reduced by sprouting in white kidney bean, faba beans, and chickpeas (Rubio et al., 2002). The practice of consumption of sprouted grains has been increased for few decades. In addition to light and temperature, seed sprouting is also greatly affected by growing media (Dharmveer et al., 2016; Park et al., 2020).
Germination of lotus seed is an effective way to enhance its nutritional value. The crude protein and lipid contents of the endosperm substantially are increased, ash content remains unchanged, phytic acid content is significantly increased, and tannin and catecholamine levels are significantly decreased by the germination of lotus seeds. On the other hand, the levels of total phenols, total flavonoids and phenolic alkaloids in lotus seeds are significantly increased after germination (Purintraphiban and Xia, 2012). The objective of this study was to investigate the nutritional value and antioxidant properties of the lotus sprouts germinated in different growing media.
Materials and Methods
Chemicals and seed materials
Folin-Ciocalteu phenol reagent; 1,1-diphenly-2-picrylhydrazyl (DPPH); 2,2'-Azino-bis (3-ethylbenzothiazoline-6-sulphonic acid) (ABTS); and pyrogallol were purchased from Sigma-Aldrich (Sigma-Aldrich Corp, St. Louis, MO, USA) and amino acid standards were obtained from Wako (Wako Pure Chemical Industries, Ltd., Osaka, Japan). All the chemicals used in this study were of analytical grade. Lotus (Nelumbo nucifera Gaertn.) seeds were bought from a local market in Daegu, Korea.
Cultivation of lotus sprouts
Twenty-five grams of intact lotus seeds (in three replications for three treatments each) were rinsed with tap water and were dipped in tap water for 2 h before kept for sprouting. The sprouts were named on the basis of the media in which they were grown, such LSSG for the lotus sprouts grown using a soybean sprouting machine (in which, the sprouts were sprinkled with tap water for 1 min every 2 h), LSSC for the lotus sprouts grown in soil kept in a container, and LSMC for the sprouts grown in mud kept in a container. The sprouts were grown at room temperature (20 ± 2℃) for 10 d.
Measurement of growth parameters and preparation of sprout powder samples
Sprout yields (total weight of lotus sprouts) in each batch were measured every day until 10 d. The randomly selected 20 sprouts from each treatment and replication were considered for the measurements of the weights of cotyledons and hypocotyls and the length of hypocotyls at 10 d.
The freshly harvested sprouts were kept at -70℃ for 24 h before freeze drying. The freeze-dried sprout samples were ground into powder using a commercial grinder (HIL-G-501, Hanil Co., Seoul, Korea) and filtered through a 100-mesh sieve.
Color measurement
The Hunter’s color values of the powdered samples were measured on the basis of ‘L’ (lightness), ‘a’ (redness), and ‘b’ (yellowness) values using a Chroma Meter (CR-300, Minolta Corp., Tokyo, Japan). A calibration plate (Minolta Corp.; YCIE = 94.5, XCIE = 0.3160, YCIE = 0.330) and a standard plate (Hunter Associates Laboratory Inc., Reston, VA, USA; ‘L’= 97.51, ‘a’= -0.18, ‘b’= 1.67) were considered for standardizing the instrument with D65 illuminant as described earlier (Kim et al., 2014).
Determination of total polyphenol content
The total polyphenol contents of the sprout samples were assayed according to the Folin-Ciocalteau method (Singleton, 1999) as described by Dhungana et al. (2015). One gram of sprout powder was extracted in 10 mL of absolute methanol using a shaking incubator (150 rpm, 25℃) for 8 h and the mixture was centrifuged (3000 rpm, 15 min). The supernatant was filtered through a 0.2-㎛ syringe filter (Waters Co.) and the filtrate extract was used for different analyses. Fifty microliters of the methanolic extract and 1000 μL of 2% (w/v) aqueous Na2CO3 were thoroughly using a vortexer and allowed to react for 3 min. After 3 min of incubation, 50 μL of 1N Folin-Ciocalteau reagent was put into the mixture and allowed to react at room temperature for 30 min under dark condition. The absorbance value of mixture was read at 750 ㎚ using a Microplate Spectrophotometer (Multiskan GO; Thermo Fisher Scientific Oy, Vantaa, Finland). The calibration curve was plotted using gallic acid (GA) as a standard. Total polyphenols contents in the samples were estimated as GA equivalents (㎍ GAE/g powder).
Determination of Free amino acid content
Free amino acid profile of the sprouts was determined following the method described by Je et al. (2005) and Kim et al. (2016) with some modifications. One milliliter of the sample extract was hydrolyzed with 10 mL of 6 N hydrochloric acid in a sealed-vacuum ampoule at 110℃ for 24 h. The hydrochloric acid was removed using a rotary evaporator and 0.2 M sodium citrate buffer (pH 2.2) was added to the remnant to make the volume 5.0 mL. The reaction mixture was passed through a cartridge (C18 Sep-Pak, Waters Co., Milford, MA, USA) and through a 0.22-㎛ filter (Millipore, Billerica, MA, USA). Amino acid content was measured using an automatic amino acid analyzer (Biochrom-20, Pharmacia Biotech, Uppsala, Sweden).
Determination of DPPH radical scavenging activity
The DPPH free radical scavenging activities of the sprout samples were measured following the method described earlier (Blois, 1958; Kim et al., 2017). Equal volumes of sample extract (0.1 mL) and freshly prepared 0.1% (w/v) methanolic solution of DPPH (0.1 mL) were mixed in microplate wells and kept at room temperature for 30 min under dark condition. After the 30 min of incubation, the absorbance values of the reaction mixtures were measured at 517 ㎚ using a microplate spectrophotometer (Multiskan GO, Thermo Fisher Scientific). The mixtures of 0.1 mL of methanol and sample extract each and the same amounts of methanol and DPPH solution were considered as control and blank, respectively. The free radical scavenging activities were estimated using the absorbance values of the different reaction mixtures as follows.
where A, Ab, and Ao are the absorbance values of the sample extracts with DPPH, control, and blank, respectively.
Determination of ABTS radical scavenging activity
The ABTS radical scavenging activities of sample extracts were measured following the method described by Miller et al. (1993) with some modifications. The ABTS cation radical was generated by mixing 2.4 mM potassium persulfate with 7 mM ABTS solution and kept for 16 h under dark condition. The reaction mixture was diluted with double-distilled water to obtain 0.7± 0.02 absorbance value at 734 ㎚. The sample extracts (20 μL) and ABTS reagent were mixed and incubated under dark condition for 30 min and then the absorbance values were read at 734 ㎚ using a microplate spectrophotometer (Multiskan GO, Thermo Fisher Scientific). The ABTS radical scavenging activity was determined using the following equation.
where AC = absorbance of ABTS radical solution alone and AS = absorbance of the mixture of ABTS radical solution and sample extracts.
Determination of superoxide dismutase (SOD)-like activity
The SOD-like activities of sample extracts were determined following the method described by Debnath et al. (2011) and Adhikari et al. (2019). A reaction mixture was prepared mixing 1.3 mL of Tris-HCl buffer (50 mM Tris, 10 mM EDTA, pH 8.5), 0.1 mL of 7.2 mM pyrogallol, and 0.1 mL of sample extracts and then incubated at 25℃ for 10 min. The reaction was terminated by mixing 0.05 mL of 1 N HCl into the mixture. The amount of pyrogallol oxidized during the reaction was determined by measuring the absorbance at 420 ㎚ using a microplate spectrophotometer (Multiskan GO, Thermo Fisher Scientific). The SOD-like activity was determined using the following equation.
Statistical analysis
Analysis of variance was conducted using SAS 9.4 (SAS Institute, Cary, NC, USA) and the significance differences between sample means were determined using the Tukey test at 5% probability. Average values of three replications are reported unless otherwise mentioned.
Results
Germination rate and sprout length
Significant differences were found in the rate of seed germination (Table 1) and sprout length (Table 2) at 5 and 10 days under different cultivation conditions. One hundred percent seeds were germinated under LSMC, whereas up to 80 and 90% seeds were germinated in LSSG and LSSC, respectively, during the cultivation period.
Table 1.
Germination rate (%) of sprouted lotus seed under different cultivations methods
Cultivation time (days) | Cultivation method | ||
LSSGz | LSSCy | LSMCx | |
5 | 75.0 ± 3.2bw | 80.0 ± 2.3b | 100 ± 0a |
10 | 80.0 ± 2.0c | 90.0 ± 1.8b | 100 ± 0a |
zLSSG: Lotus sprout cultivated using soybean grower, yLSSC: Lotus sprout cultivated in soil kept in a container, xLSMC: Lotus sprout cultivated in mud kept in a container. wValues are means ± SD of triplicate measurements. The values followed by different letters in the same row are significantly different (p < 0.05).
The longest sprout was obtained in LSMC (4.79 and 26.79 ㎝) followed by LSSC (1.95 and 5.4 ㎝), and LSSG (0.60 and 2.85 ㎝) at 5 and 10 days, respectively. More than 8 and 9 times longer sprouts were obtained at 5 and 10 days, respectively, in LSMC than in LSSG (Table 2).
Table 2.
Total length (㎝) of lotus sprouts under different cultivation methods
Cultivation time (days) | Cultivation method | ||
LSSGz (㎝) | LSSCy (㎝) | LSMCx (㎝) | |
5 |
0.60 ± 0.02cw (100)v |
1.95 ± 0.15b (327) |
4.79 ± 1.23a (805) |
10 |
2.85 ± 0.18c (100) |
5.40 ± 1.29b (189) |
26.79 ± 3.44a (940) |
zLSSG: Lotus sprout cultivated using soybean grower, yLSSC: Lotus sprout cultivated in soil kept in a container, xLSMC: Lotus sprout cultivated in mud kept in a container. wValues are means ± SD of triplicate measurements. The values followed by different letters in the same row are significantly different (p < 0.05). vValues in parentheses represent relative length of sprouts expressed in percentage of LSSG.
Color
Several parameters of the Hunter’s color values of the different parts of lotus sprouts were significantly different (Table 3). A higher yellowness value for husk was obtained in LSSC (7.16) than that in LSSG (6.50), whereas lightness and redness values of husk were not significantly different among different methods of sprout cultivation at 10 d. The L*, a*, and b* values of cotyledons under LSMC were significantly higher than those under LSSG. The highest redness value of hypocotyl was found under LSSG (-3.96) but the lightness values of hypocotyl was the lowest under LSSG (48.03) compared to that of LSSC (-5.79 and 53.92) and LSMC (-5.97 and 54.88), respectively.
Table 3.
Hunter’s color value of lotus sprouts cultivated for 10 days under different cultivation methods
zLSSG: Lotus sprout cultivated using soybean grower, yLSSC: Lotus sprout cultivated in soil kept in a container, xLSMC: Lotus sprout cultivated in mud kept in a container. wL; lightness (100, white; 0, black), a; redness (‒, greenness; +, redness), b; yellowness (‒, blueness; +, yellowness). vValues are means±SD of triplicate measurements. The values followed by different letters in the same row are significantly different (p < 0.05).
Free amino acid composition
The amounts of different essential and non-essential amino acids measured in cotyledons and hypocotyls of lotus sprouts grown under different conditions are presented in Table 4. Higher amounts of total free amino acids were found in cotyledons (33.96, 21.45, and 38.90 ㎎/g) than in hypocotyls (15.77, 7.90, and 15.29 ㎎/g) for LSSG, LSSC, and LSMC, respectively. The ratios of total essential to total non-essential amino acids were higher in hypocotyls (0.36, 0.31, and 0.46) than in cotyledons (0.34, 0.25, and 0.40), respectively.
Table 4.
Free amino acid composition (㎎/g of dry weight) of lotus sprouts cultivated for 10 days under different cultivation methods
Antioxidant potential
The antioxidant potentials of different parts of lotus sprouts were evaluated through parameters like DPPH and ABTS free radical scavenging potentials, SOD-like activities, and total polyphenol content. Most of the parameters in the husk, cotyledon, and hypocotyl were significantly different with the growing media (Fig. 1). The DPPH and SOD-like activities of the hypocotyls of LSMC (71.25 and 71.46%) were highest among different parts of sprouts grown in three media. The hypocotyl of LSSC (57.36%) showed highest ABTS radical scavenging potential. Similarly, the total polyphenol content of the hypocotyl of LSMC (50.33 ㎍ GAE/g) was the highest and that of the husk of LSSG (24.08 ㎍ GAE/g) was the lowest.
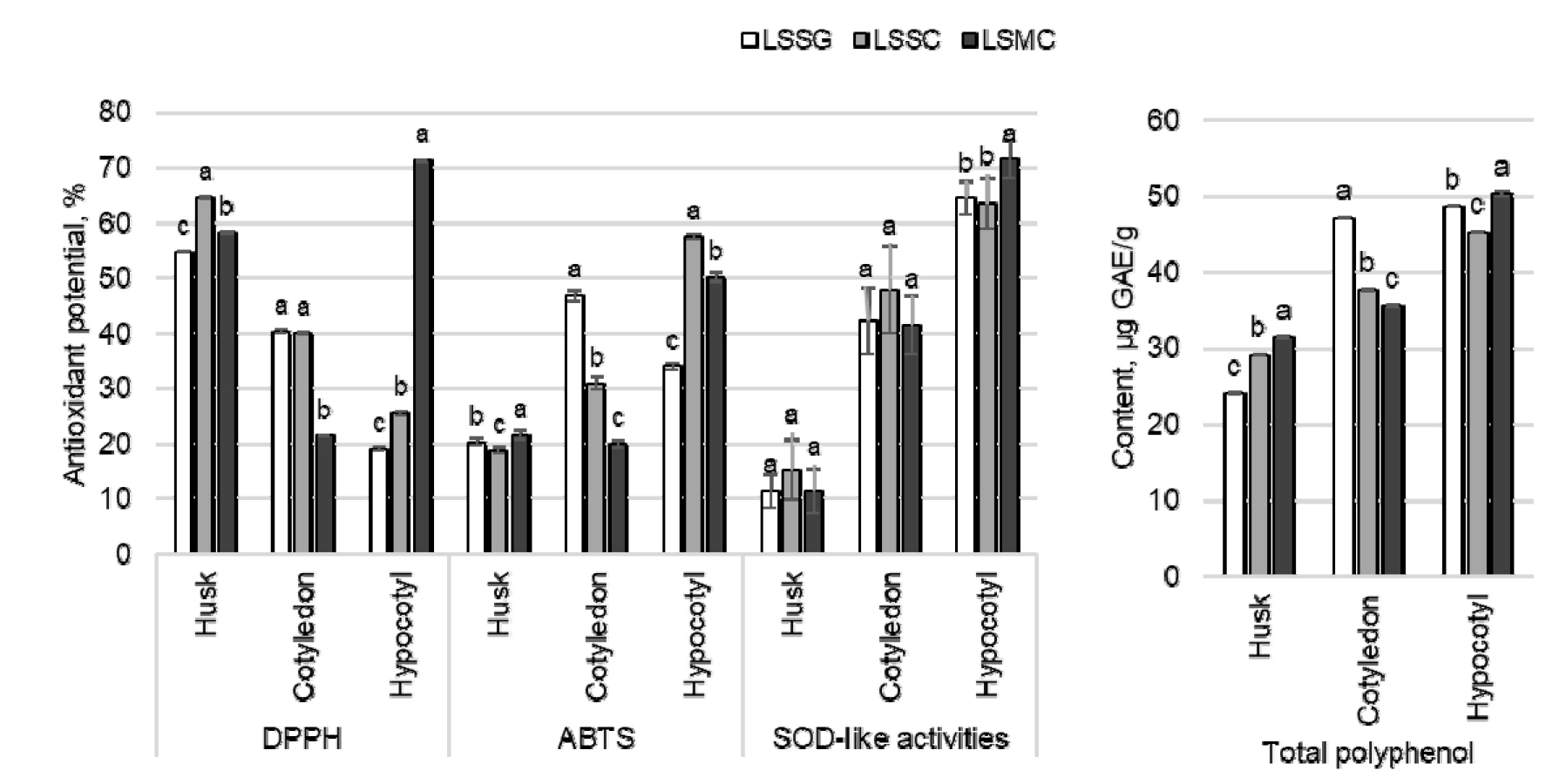
Fig. 1
Changes in DPPH and ABTS free radical scavenging potential, SOD-like activities, and total polyphenol content of lotus sprouts cultivated for 10 days under different cultivation methods. LSSG: Lotus sprout cultivated using soybean grower, LSSC: Lotus sprout cultivated in soil kept in a container, LSMC: Lotus sprout cultivated in mud kept in a container. GAE: Gallic acid equivalent. Different letters above the bar diagrams of the same parts of sprout of the same antioxidant category are significantly different (p < 0.05). The vertical line in the bars show standard deviation (n = 3).
Discussion
The variation in germination and growth of sprout samples might be owing to the difference in growing media (Mathowa et al., 2014). Color of any food product may substantially affect consumers’ preference towards the food. The significant discrepancies in the color value of the sprout samples might be due to the variation in the phytochemical accumulation in the samples.
Free amino acid content of sprouts may vary in different parts. The total free amino acid content was more than 3 times higher in hypocotyls than in cotyledons of okra sprouts (Jeon et al., 2017). Foods containing higher ratios of essential to non-essential amino acids are regarded as the foods with well-balanced protein content (Reeds, 2000). Glutamic acid and γ-Amino-n-butyric acid (GABA) were some of the major amino acids in the sprout samples. GABA is principally produced in plants by decarboxylation of glutamic acid in the presence of glutamate decarboxylase (Nikmaram et al., 2017). GABA and glycine are found to have roles in enhancing learning and memory, against stroke and neurodegenerative diseases; relieving anxiety, sedation, anticonvulsant, and muscle relaxation functions (Krogsgaard-Larsen, 1989; Mody et al., 1994; Oh and Oh, 2004). The GABA-rich foods are also regarded as brain foods and play roles in correcting several bioactive functions, such as blood cholesterol level, blood pressure, cerebral blood flow, insomnia, depression, and pain (Dhakal et al., 2012). GABA also shows anti-diabetic effect (Nikmaram et al., 2017).
The variation in the nutrient content and antioxidant potentials of the sprout samples might be due to the difference in growing media (Mathowa et al., 2014) as well. Although the reason was not well known, overall results showed that the antioxidant potential of the cotyledons produced in sprout grower was higher, whereas that of the hypocotyls grown in mud was higher than in other cultivation conditions. Overall, the antioxidant potential of hypocotyl was higher than that of husk and cotyledon of lotus sprouts. Similar results of higher antioxidant potential in the hypocotyl of peanut sprouts was found in a previous report (Adhikari et al., 2018). Free radicals are produced in the body as a result of normal cell metabolisms as well as due to the impact of external factors, including stresses. When the level of free radicals is not adequately scavenged by the antioxidants, the accumulation of free radicals in the body results in oxidative stress. Oxidative stress plays a major role in the development various diseases, including cancer, autoimmune disorders, aging, cataract, rheumatoid arthritis. Antioxidants, which are either naturally produced in the body or supplied through foods, play vital roles in preventing and controlling the ill-effects caused by the oxidative stress (Pham-Huy et al., 2008). Plant polyphenols are good antioxidants for good health (Pandey and Rizvi, 2009). Several enzymatic and non-enzymatic antioxidants, including glutathione peroxidase, superoxide dismutase, carotenoids, and polyphenols account for antioxidant activities (Kurutas, 2015). Similarly, a number of factors, such as oxidation environments, segregating characteristics of specific antioxidants, and the situation of oxidizable substrate collectively govern the antioxidant potential of foods (Frankel and Meyer, 2000). Therefore, a visible increase in the quantity of a specific antioxidant may not always determine higher antioxidant activity of a sample.
Conclusively, the physicochemical properties and antioxidant potential of lotus sprouts grown in sprouting machine (LSSG), soil (LSSC), and mud (LSMC) were evaluated. The highest seed germination rate and longest sprouts were found in LSMC followed by LSSC and LSSG. The cotyledons had higher amount of total free amino acids as well as the ratio of total essential to total non-essential amino acids than in cotyledons. Similarly, the total polyphenol content of the hypocotyl of LSMC was higher than the other samples. The results indicated that the lotus sprouts grown in mud could be a good source of nutrition and natural antioxidants.