Introduction
Materials and methods
Materials
Sample preparation
Cell culture and treatment
Cell viability assay
Measurement of NO production
Western blot analysis
Reverse transcriptase-polymerase chain reaction (RT-PCR)
Statistical analysis
Results and Discussion
The effect of QM-B on NO production and iNOS, COX-2 and IL-6 expression in LPS-induced RAW264.7 cells
The effect of QM-B on NF-κB signaling activation in LPS-induced RAW264.7 cells
The effect of QM-B on MAPK signaling activation in LPS-induced RAW264.7 cells
The effect of QM-B on HO-1 activation in RAW264.7 cells
Introduction
Inflammation is protective response of tissues to harmful irritate, such as injury and microbes. Under pathophysiological conditions, acute or chronic inflammation may cause various inflammatory diseases such as diabetes, arthritis, septic shock and cancer. The macrophages produce preventative intermediates such as interleukin 6 (IL-6) interleukin 1β (IL-β), tumor necrosis factor α (TNF-α) and nitrogen oxides (NO), which play a pivotal role in the inflammatory process and also serve as a host defense mechanism. NO has been reported to activate nuclear factor kappa B (NF-κB) in peripheral blood monaryotic cells, which is a transcription factor in the expression of the inducible nitric oxide synthases (iNOS) gene in response to inflammation (Tripathi et al., 2007; Wang et al., 2018). Heme oxygenase 1 (HO-1) is induced by various stimuli, such as proinflammatory cytokines and heavy metals. Expression of HO-1 has been reported to provide an important protective response of cells against oxidative damage, because HO-1 expression may decrease the cellular heme level and elevate the level of bilirubin, which is demonstrated a potent scavenging activity against reactive oxygen species (Doi et al., 1999; Fang et al., 2003).
Quercus mongolica (QM) is a species of oak native to Korea. QM has been reported to exert anti-oxidation, anti-microbial, and anti-allergic activities as an oriental traditional medicine. The components of leaves and bark of QM are known to be flavonoids, phenols, tannins and triterpenoid (Kong et al., 2001a; Kong et al., 2001b; Yeo et al., 2008; Bak et al., 2011; Yin et al., 2019) There are many research on leaves and barks of QM, but little is known about branches (QM-B). Therefore, we evaluates the anti-inflammatory effect of QM-B in LPS-induced RAW264.7 cells and elucidates the mechanism of NF-κB and mitogen activated protein kinase (MAPK) regulation and HO-1 expression as the molecular target.
Materials and methods
Materials
Dulbecco’s Modified Eagle medium (DMEM)/F-12 1:1 Modified medium (DMEM/F-12) was purchased from Lonza (Walkersville, MD, USA). lipopolysaccharide (LPS) and 3-(4,5-dimethylthiazol-2-yl)-2,5-diphenyltetrazolium bromide (MTT) were purchased from Sigma-Aldrich (St. Louis, MO, USA). Antibodies against phospho-IκB-α, IκB-α, phospho-extracellular signal-regulated kinase1/2 (p-ERK1/2), ERK1/2, phospho-p38 (p-p38), p38, HO-1 and β-actin were purchased from Cell Signaling technology (Bervely, MA, USA).
Sample preparation
The branches of Q. mongolica (QM-B, specimen number : FMCQm-P-20190521-005~009) were collected from Wolhak-ri, Buk-myeon, Inje-gun, Kangwon-do. 10g of dried QM-B were extracted with 200 mL of 70% ethanol for 72 hours under shaking at the room temperature. After 72 hours, the ethanol-soluble fraction was filtered and concentrated a rotary vacuum evaporator. The ethanol extracts from the QM-B were kept in a refrigerator until use.
Cell culture and treatment
RAW264.7 cells were purchased American Type Culture Collection (ACTT, Virginia, USA) and grown in Dulbecco's Modified Eagle (Lonza, Walkerscille, MD, USA) supplemented with 10% fetal bovine serum (FBS), penicillin (100 units/mL) and streptomycin (100 ㎍/mL) under an atmosphere of 5% CO2 at 37℃. QM-B was dissolved in dimethyl sulfoxide (DMSO) and treated to cells. DMSO was used as a vehicle and the final DMSO concentration did not exceed 0.1% (v/v).
Cell viability assay
RAW264.7 cells viability were estimated by determining mitochondrial reductase function with the MTT assay. Briefly, RAW264.7 cells (3 × 104 cells/well) were cultured in 12-well plate and incubated for 24 hours. The RAW264.7 cells were treated with QM-B at the indicated concentrations for 24 hours. Then, the cells were cultured with 200 μL of 1 ㎎/mL MTT solution for an additional 2 hours. The resulting crystals were dissolved in DMSO. The formazan synthesised was dissolved in DMSO and the optical density was measured at 570 ㎚ using UV/Visible spectrophotometer (Perkin Elmer, Nowolk, CT, USA).
Measurement of NO production
RAW264.7 cells were cultured in 12-well plate for overnight. The cells were pretreated with QM-B at the indicated concentrations for 6 hours and then co-treated with LPS (1㎍/mL) for 18 hours. NO levels were estimated by Griess assay. Briefly, 50 μL of the cell culture supernatants were mixed with 50 μL of Griess reagent (Sigma Aldrich, St. Louis, MO, USA) and followed by reaction for 15 minutes at the room temperature. After 15 minutes, absorbance values were measured using a UV/Visible spectrophotometer (Perkin Elmer, Norwolk, CT, USA) at 540 ㎚.
Western blot analysis
The cells were harvested in ice-cold 1XPBS, and lysed in 1X radioimmunoprecipitation assay (RIPA) buffer (Cell signaling, Bervely, MA, USA) supplemented with protease inhibitor cocktail and phosphatase inhibitor cocktail, and centrifuged at 15,000 rpm for 10 minutes at 4℃. The protein content of the cell lysates was then determined using bicinchoninic acid (BCA). Protein in each sample were resolved by 10% Sodium dodecyl sulphate polyacrylamide gel electrophoresis (SDS-PAGE), transferred to the nitrocellulose membranes (Bio-Rad, CA, USA). The membranes were blocked for non-specific binding with 5% skim milk in Tris-buffered saline containing 0.05% Tween 20 (TBS-T) for 1 hour at room temperature. The membrane was incubated with diluted primary antibodies for proper times and then washed three times with TBS-T and further incubated with secondary antibody for 1 hour at room temperature. The chemiluminescence was detected with ECL Western blotting substrate and visualized in Chemi Doc MP Imaging system (Bio-rad, CA, USA).
Reverse transcriptase-polymerase chain reaction (RT-PCR)
Total RNA was prepared using a RNeasy Mini Kit (Qiagen, Valencia, CA, USA) and total RNA (1 ㎍) was reverse-transcribed using a Verso cDNA Kit (Thermo Scientific, Pittsburgh, PA, USA) according to the manufacturer’s protocol for cDNA synthesis. PCR was carried out using PCR Master Mix Kit (Promega, Madison, WI, USA) with primers for mouse iNOS, mouse COX-2, mouse IL-6 and mouse GAPDH (Table 1.).
Table 1. The primer sequences used in this study
Statistical analysis
Data are expressed as mean ± standard deviation (SD). Each experiment was repeated at least three times. Statistical analysis was performed with one-way ANOVA followed by Dunnett's test. Differences with *P or #P < 0.05 were considered statistically significant.
Results and Discussion
The effect of QM-B on NO production and iNOS, COX-2 and IL-6 expression in LPS-induced RAW264.7 cells
Nitric oxide synthases (NOS) synthesize the metastable free radical NO. NO is an important mediator and regulator of various processes in the immune, cardiovascular and nervous systems. Also, NO is implicated in pathophysiologic states as diverse as hypertension, septic shock, stroke, and neurodegenerative diseases (Ignarro, 1990; Davies et al., 1997; Hou et al., 1999). During the inflammatory process, large amounts of the pro-inflammatory mediators, NO and prostaglandin E2 (PGE2) are generated by the iNOS and cyclooxygenase-2 (COX-2) (Kim et al., 2007; Wang et al., 2019).
To determine if the QM-B could suppress NO generation by LPS, RAW264.7 cells were pretreated with the QM-B for 6 hours and then co-treated with LPS (1 ㎍/mL) for the additional 18 hours. As shown in Fig. 1A, treatment of LPS without QM-B induced NO overproduction in LPS-induced RAW264.7 cells, while pretreatment of QM-B suppress LPS mediated NO overproduction. As shown in Fig. 1B, QM-B maintained the cell viability. Since NO production is regulated by iNOS, COX-2 and IL-6 expression, the effect of QM-B on iNOS, COX-2 and IL-6 expression was performed by western blot and RT-PCR. As shown in Fig. 1C, the treatment of QM-B attenuated the mRNA expression of iNOS, COX-2 and IL-6. Thus, QM-B blocked the over- production of NO by suppressing LPS-induced iNOS, COX-2 and IL-6 over-expression in RAW264.7 cells.
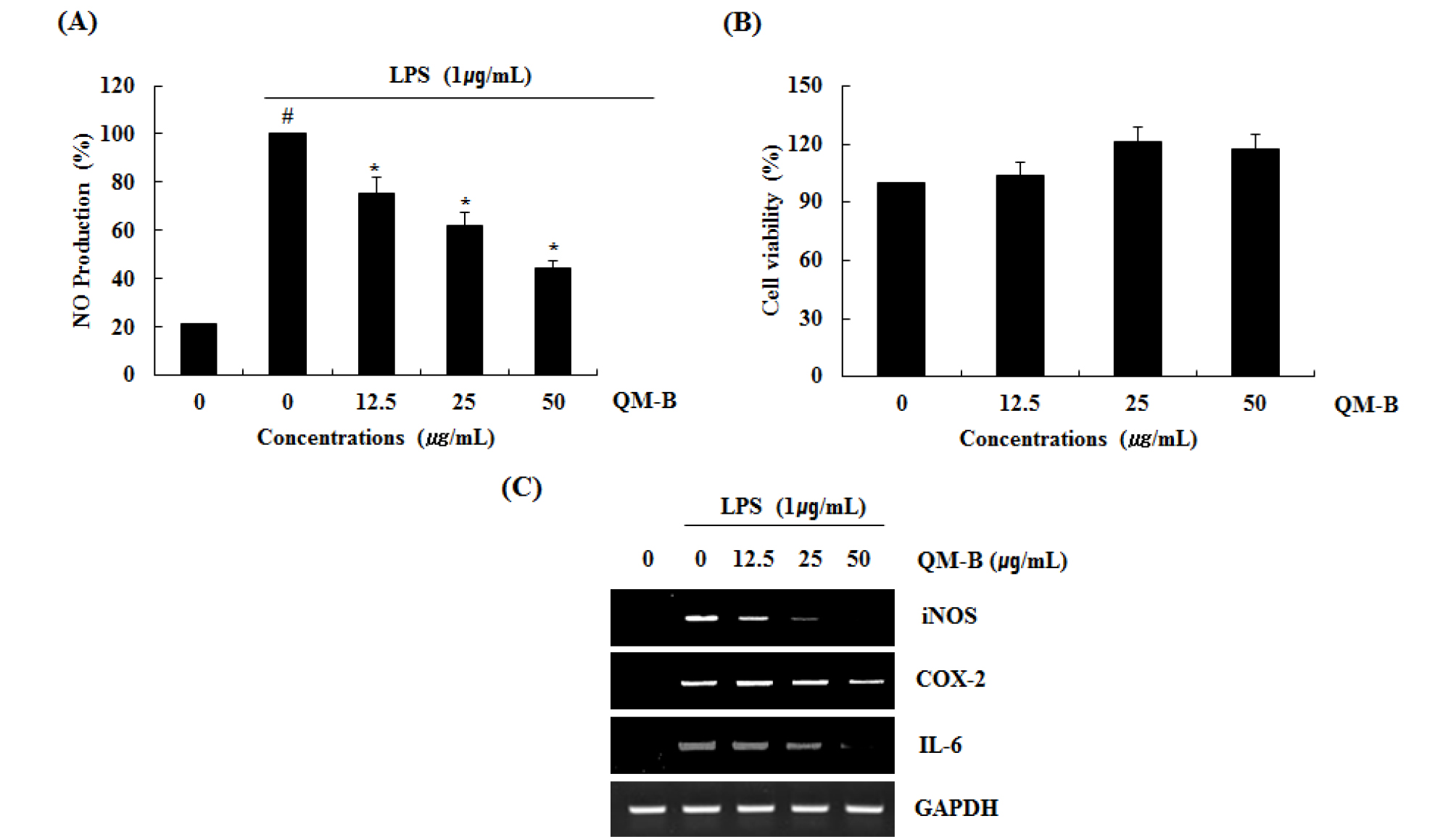
Fig. 1.
The effect of QM-B on NO production and iNOS, COX-2 and IL-6 expression in LPS-stimulated RAW264.7 cells. (A) RAW264.7 cells were pre-treated with QM-B at the indicated concentrations for 6 hours and then co-treated with LPS (1 ㎍/mL) for the additional 18 hours. After treatment, NO production was measured using the media and Griess reagent. (B) RAW264.7 cells were treated with QM-B at the indicated concentrations for 24 hours. Cell viability was measured using MTT assay system and expressed as % cell viability. (C) For RT-PCR, RAW264.7 cells were pre-treated with QM-B at the indicated concentrations for 6 hours and then co-treated with LPS (1 ㎍/mL) for the additional 18 hours. Total RNA was isolated and RT-PCR was performed for iNOS and COX-2 and IL-6. Values given are the mean ± SD (n = 3). *P < 0.05 compared to LPS treatment without QM-B. GAPDH was used as an internal control for RT-PCR.
The effect of QM-B on NF-κB signaling activation in LPS-induced RAW264.7 cells
NF-KB is one of the most key transcription factors and is found in cytokines, growth factors, chymokins, cell adhesives, and cell types that express some acute-phase proteins in healthy and diseased conditions. NF-KB plays a crucial role in the regulation of cell survival genes and is known to coordinate the expression of cytokine with proinflammatory enzymes such as iNOS, COX-2, IL-6 and TNF-α. NF-KB is located in the cytoplasm as an inactivated complex bound to IKB-α, which is phosphorylated and degradaded, and then dissociates to produce activated NF-KB (Kaibori et al., 1999; Kim et al., 2007; Li et al., 2017; Ran et al., 2018; Woo et al., 2018).
To elucidate the effect of QM-B on NF-κB signaling activation, we performed a western blot for degradation and phosphorylation of IκB-α in LPS-stimulated RAW264.7 cells. As shown in Fig. 2, LPS induced subsequent degradation and phosphorylation of IκB-α at 30 minutes after the stimulation. However, pretreatment of QM-B blocked LPS-induced IκB-α degradation in a dose-dependent manner.
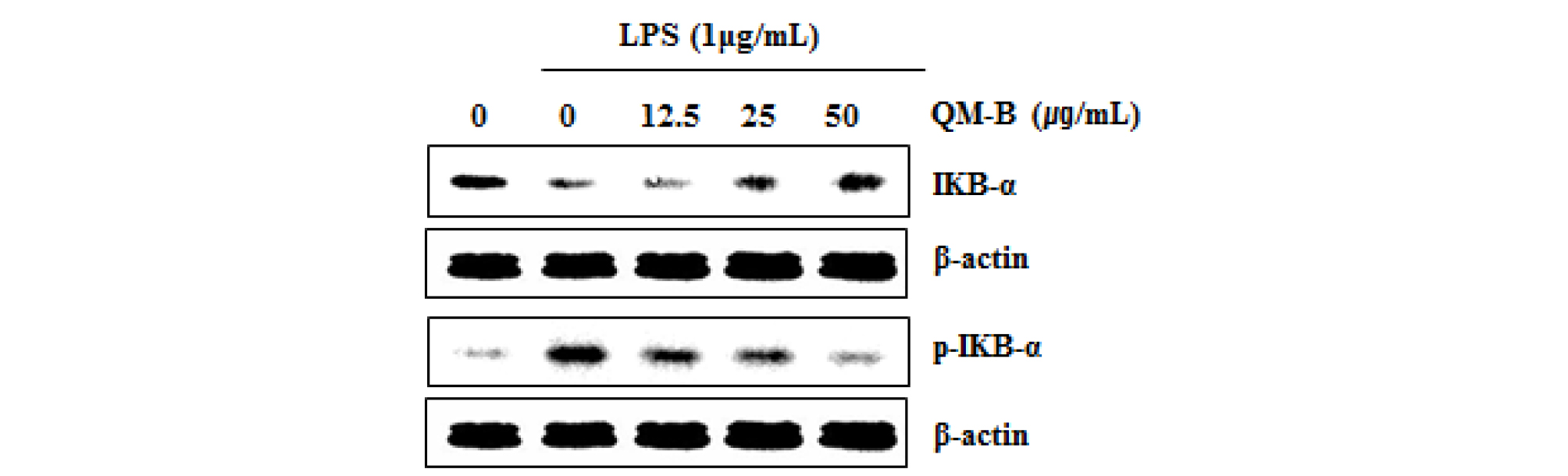
Fig. 2.
The effect of QM-B on NF-κB signaling activation in LPS-stimulated RAW264.7 cells. (A) RAW264.7 cells were pretreated with QM-B for 6 hours and then co-treated with LPS (1 ㎍/mL) for 30 minutes. For Western blot analysis, the cell lysates were subjected to SDS-PAGE and the Western blot was performed using antibodies against p-IκB-α and IκB-α. β-actin was used as internal control.
The effect of QM-B on MAPK signaling activation in LPS-induced RAW264.7 cells
MAPK plays an important role in regulating cell growth and differentiation and controlling cellular responses to cytokines and stress. MAPK are involved in the LPS-induced iNOS expression signaling pathway (Kim et al., 2007). There is growing evidence that activation of MAPK by phosphorylating ERK1/2, p38 and JNK mediates excessive production of the pro-inflammatory mediators, which results to the initiation and development of inflammatory disease (Park et al., 2018; Ran et al., 2018). Also, MAPK has considered as a typical molecular target for the development of anti- inflammatory agents. To further investigate whether decrease of NF-κB activation by QM-B treatment is associated with the regulation of p38 and ERK1/2 activation, we evaluated the effects of QM-B on phosphorylation of p38 and ERK1/2 in LPS-induced RAW264.7 cells. As shown in Fig. 3, Increase of p38 and ERK1/2 phosphorylation was observed in RAW264.7 cells by LPS. However, QM-B suppressed phosphorylation of p38 and ERK1/2, indicating that QM-B suppress the inflammatory response by inhibiting p38 and ERK1/2 activation in LPS-induced RAW264.7 cells.
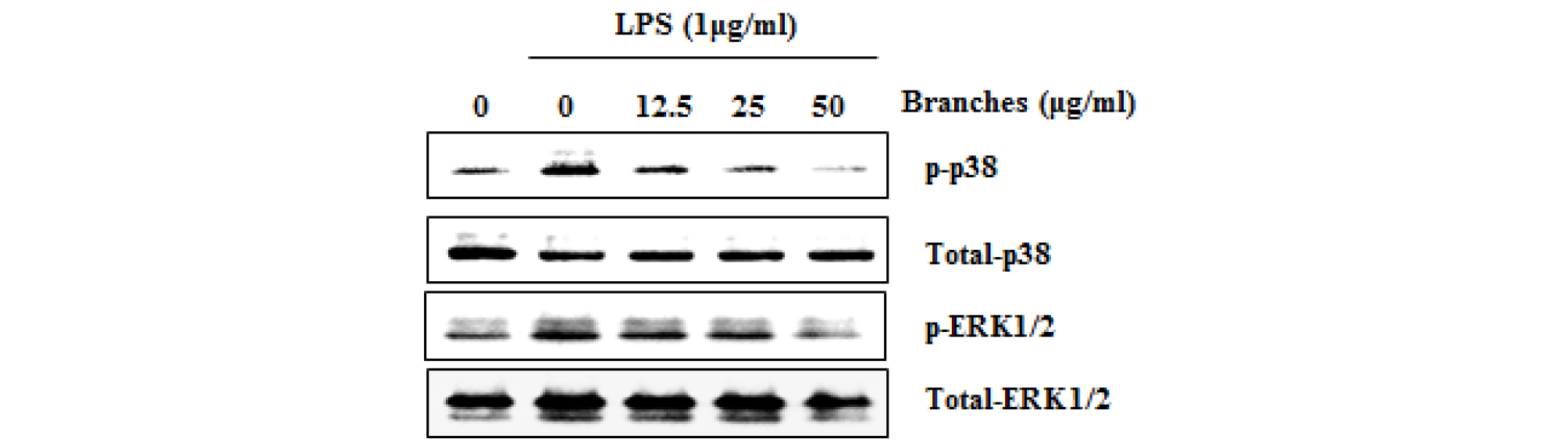
Fig. 3.
The effect of QM-B on MAPK signaling activation in LPS-stimulated RAW264.7 cells. RAW264.7 cells were pretreated with QM-B for 6 hours and then co-treated with LPS (1 ㎍/mL) for 30 minutes. For Western blot analysis, the cell lysates were subjected to SDS-PAGE and the Western blot was performed using antibodies against p-ERK1/2, p-p38 and Total p-38 and Total ERK1/2 were used as internal control.
The effect of QM-B on HO-1 activation in RAW264.7 cells
HO-1 is an enzyme that promotes the oxidation degradation of heme, forming bilburdine, carbon monoxide, and free iron. The evidence represented an important role of HO-1 in cell protection and other more diverse biological functions. The high expression of HO-1 occurs in various tumors, and HO-1 is known to play an important role in rapid tumor growth because of its anti-oxidant and anti-apoptotic effects. Induction of HO-1 expression protects against the cytotoxicity of oxidative stress and apoptotic cell death (Eyssen-Hernandez et al., 1996; Doi et al., 1999; Jung et al., 2006; Paine et al., 2010). In this study, our data showed that QM-B increased HO-1 expression (Fig. 4A and B), and inhibition of HO-1 by the treatment of PD98059 (ERK inhibitor) (Fig. 4C) or SB230580 (p38 inhibitor) (Fig. 4D). These findings indicate that HO-1 may be a potential target for the anti-inflammatory effect of QM-B.
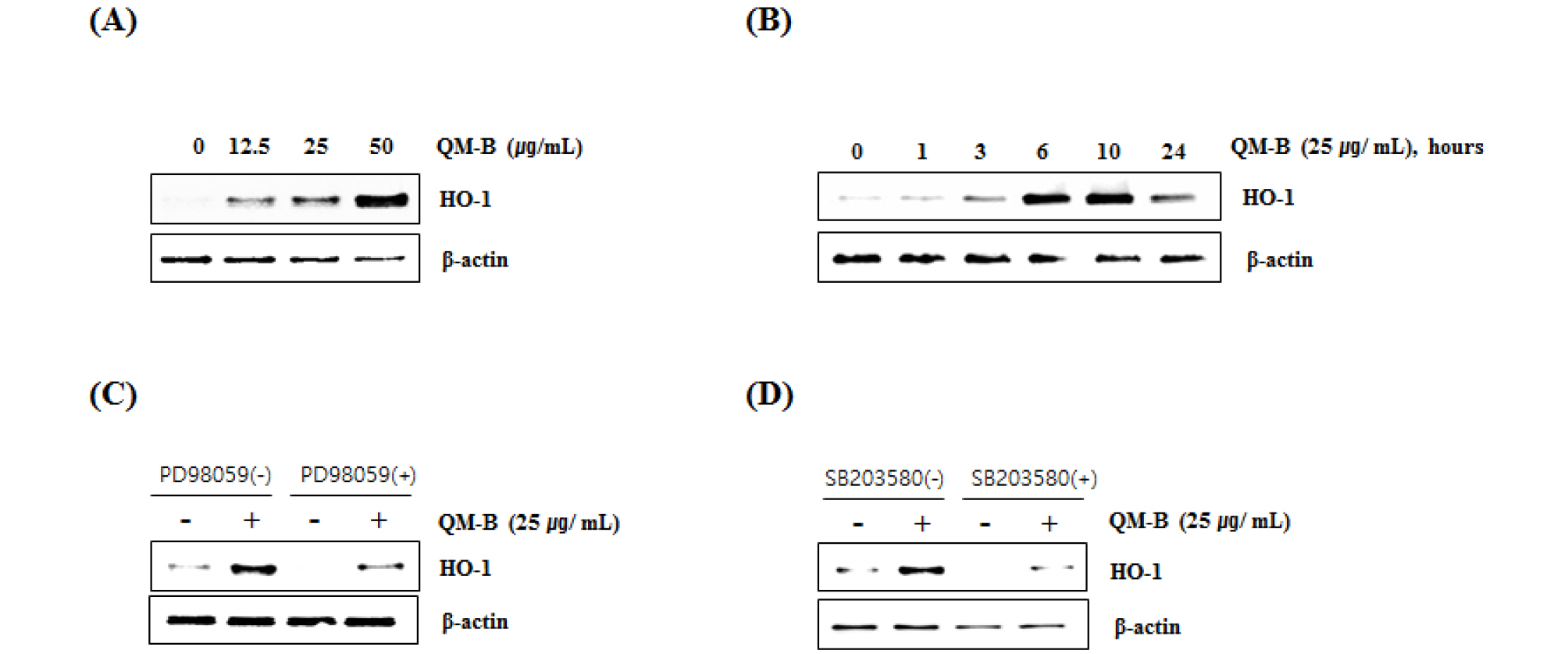
Fig. 4.
Effect of QM-B on HO-1 expression in RAW264.7 cells. (A) RAW264.7 cells were treated with QM-B at the indicated concentrations for 24 hours. (B) RAW264.7 cells were treated with 25 ㎍/mL of QM-B for the indicated times. For Western blot analysis, the cell lysates were subjected to SDS-PAGE and the Western blot was performed using antibody against HO-1. (C) RAW264.7 cells were treated with PD98059 (20 μM, ERK1/2 inhibitor) or SB203580 (20 μM, p38 inhibitor) for 2 hours, and then co-treated with QM-B for 10 hours. For Western blot analysis, the cell lysates were subjected to SDS-PAGE and the Western blot was performed using antibody against HO-1. β-actin was used as internal control for Western blot analysis.
Taken together, these results demonstrated that QM-B blocked NO production via inhibiting iNOS, COX-2 and IL-6 expression through suppressing the activation of NF-κB and MAPK signaling pathway. In addition, the induction of HO-1 expression by QM is involved in the anti-inflammatory effect of QM-B. These findings suggest that QM-B may have great potential for the development of anti-inflammatory drug to treat acute and chronic inflammatory disorders.