Introduction
Materials and Methods
Plant material and treatment
Measurement of plant growth parameters
Measurement of carotenoid content
Measurement of free amino acid and proline
Statistical analysis
Results and Discussion
Plant growth parameter
Free amino acid
Carotenoid content
Proline content
Introduction
Chinese cabbage (Brassica rapa subsp. Pekinensis) is a leafy vegetable plant belonging to the family Brassicaceae. It is an important vegetable plant that is consumed throughout the year in South Korea (Soengas et al., 2011; Kim et al., 2013). The cultivation of Brassica crops has been increased in Korea (Kim et al., 2018). Annually, approximately 2 million tons of Chinese cabbage is produced in Korea, accounting for 25% of total vegetable consumed in the country (Kim et al., 2014). Chinese cabbage is rich in calcium, vitamin C, α- and β-carotenoids, free amino acids, proline, and glucosinolates that possesses anti-carcinogenic and antioxidant properties (Wang et al., 2011; Lee et al., 2015).
In South Korea, Chinese cabbage is cultivated in open field crop that are facing frequent water stress during their growing period because of climate change and abnormal weather (Lee et al., 2017). Especially, young stage of Chinese cabbage seedlings may be severely damage depending on intensity and the duration of drought stress (Bray, 1997). Preliminary experiment showed that after drought stress induction, plant growth parameters were significantly lower in the drought treated plants than control plants. Drought treated Chinese cabbage increased glucosinolates (GLSs) contents at two weeks of drought stress (Shawon et al., 2017).
Further, drought stress has a negative effect on carotenoid synthesis in plants (Haisel et al., 2006; Zeid and Sheedeed, 2006). Carotenoid is a functional component of Chinese cabbage, and it acts as an antioxidant and defends plant cells from light by the participating in light absorption (Demmig-Adams and Adams, 1996). Amino acids are identified as precursors and biosynthesis components of GLSs, proteins, and other nitrogen-based compounds (Gomes and Rosa, 2000; Rai, 2002). Free amino acids also play important roles in the adaptation of plants to diverse habitat (Gomes and Rosa, 2000). Furthermore, it has been reported that free amino acid accumulation in plants increases under drought stress condition (Rai, 2002). Proline helps plants to overcome stress conditions, such as drought (Szabados and Savoure, 2009). Accumulation of proline in plant is influenced by drought stress (Choudhary et al., 2005).
However, to the best of our knowledge the effects of drought stress on plant growth parameters, carotenoids, free amino acid and proline accumulation in autumn growing cultivar of Chinese cabbage have not been widely studied in Korea, as well as in other parts of the world. Therefore, the aim of this study was to investigate morphological traits and changes in carotenoids, free amino acids, and proline content of autumn growing Chinese cabbage under drought stress condition.
Materials and Methods
Plant material and treatment
The experiment was undertaken in a greenhouse at the Wonkwang University, Iksan, Korea (latitude, 35°56'N; longitude, 126°57'E) during September-November, 2017. The seeds of Chinese cabbage (cv. Bulam Plus, Farm Hannong Co, Gyeonggi-do, Korea) were sown in a plug tray containing commercial growing media (Alpha-Plus; Sang-Lim Company, Iksan, South Korea). One-month-old seedlings were transplanted into plastic pots (28 ㎝ diameter × 22 ㎝ high) and maintained for up to three weeks (Fig. 1). The soil water content of drought treated and control plants were measured and maintained at 10% and 30% respectively (Fig. 2) by time-domain reflectometry (Field Scout TDR 100, Spectrum Technologies Inc, Aurora, USA). The seedlings received natural radiation and were watered daily using water can.
Measurement of plant growth parameters
At each harvest, Chinese cabbage plants were harvested at one, two, and three weeks after drought stress treatment. The number of leaves was counted manually, except those that were < 1 ㎝ in length. The plants were uprooted from pots, and the roots were carefully separated and washed with tap water. The leaf area per plant was measured using a leaf area meter (Li-3100, Li-Cor Inc., Neb., USA). The fresh weight of total leaves was measured using an electronic balance.
Measurement of carotenoid content
reeze-dried leaves of Chinese cabbage were pulverized and the lyophilized powder was used in further analysis. The content of carotenoids was determined by the methods of Tuan et al. (2012) with slight modifications. Briefly, 500 ㎎ of lyophilized powder of Chinese cabbage was mixed vigorously with 5 ㎖ of ethanol for 5 min and added to boiling water bath (75℃). Subsequently, 1.5 ㎖ of 80% potassium hydroxide was added. After 10 min of incubation under with ice cold condition, 2.5 ㎖ of ultra-pure water was added to the solution. Further, the crude sample was separated after mixing with equal volume of hexane. The organic solvent layer was centrifuged at 3000 rpm for 3 min at 4℃. The collected upper layer of the extract was evaporated at room temperature and 2 ㎖ of dichloromethane/methanol (50:50, v/v) was added to dissolve the collected organic layer. For HPLC analysis, the filtered liquid was analyzed using a Perkin Elmer Flexar equipped with a C30 YMC column (250 ㎜ length × 4.6 ㎜ diameter, 3.0 ㎛ particle size; Waters Corporation, Milford, MA). The HPLC analysis was carried out with a flow rate of 1.0 ㎖/min and the detection wavelength was measured at 454 ㎚. The column oven temperature was maintained at 40℃. The solvent system employed was (A) 25:75 (v/v) water/methanol and (B) 100% ethyl acetate. The gradient program used was as follows: 0 min, 0% B; 0-4 min, 70% B; 4-20 min, 75% B; 20-28 min, 100% B; suddenly dropped to solvent 60% B at 28.1 min, and then maintained constant with solvent 60% B for 7 min (total 35 min). Individual carotenoids were quantified as ㎎ ㎏-1 dry weight (DW) by comparing the peak area of standard lutein, zeaxanthin, α-carotene, and β-carotene, respectively.
Measurement of free amino acid and proline
The 100 ㎎ of lyophilized powder in a 2 ㎖ test tube, 1.2 ㎖ of 5% trichloroacetic acid (TCA) was added. The solution was vigorously vortexed for 5 min, incubated at room temperature for 60 min, and then centrifuged at 15,000 rpm for 15 min at 4℃. The supernatant was diluted 100 times with 0.1 M HCl and the filtered through a 0.45 ㎛ PTFE syringe filter (Advantec DISMIC-13HP; Toyo Roshi Kaisha Ltd., Tokyo, Japan). The filtrate was then analyzed by high performance liquid chromatography (HPLC; Agilent Technologies, Palo Alto, CA). The HPLC analysis of free amino acids was carried out by ‘a rapid, accurate, sensitive, and reproducible HPLC analysis of amino acids’ using Zorbax Eclipse-AAA Columns and the Agilent 1100 HPLC. Briefly, the separation of free amino acids was performed on a Zorbax Eclipse-AAA analytical (150 ㎜ length × 4.6 ㎜ internal diameter (i.d.), 5 ㎛ particle size) column with guard column Zorbax Eclipse-AAA 4-Pack (12.5 ㎜ length × 4.6 ㎜ (i.d.), 5 ㎛ particle size; Agilent Technologies). The detection wavelength was measured at 338 ㎚, and the column oven temperature was maintained at 40℃. The injection volume was 10 ㎖. The solvent system was delivered at a rate of 2.0 ㎖/min and consisted of a mixture of 40 mM NaH2PO4·H2O (pH 7.8, solvent A) and CAN:MeOH:water (45:45:10, v/v/v) (solvent B). The gradient program used was as follows: 0 min, 0% B; 0-1.9 min, 0% B; 1.9-21.1 min, 57% B; 21.1-21.6 min, 100% B; 21.6-25 min, 100% B; 25-25.1 min, 0% B; 25.1-30 min, 0% B. Further, 50 pmol/µL amino acid was used the standard. The quantification of different amino acids was based on peak area and calculated as equivalents of standard compounds. The content of amino acid is expressed as ㎎/100 g dry weight (DW).
Statistical analysis
The experimental design was conducted in a completely randomized design with 5 replications. The values are means ± SD of five measurements. SAS software version 9.3 (SAS Institute, Cary, North Carolina, USA) was used for data analysis and identify significant effect of treatments. The ANOVA procedure was applied to determine significant difference followed by Duncan’s Multiple Range Test (DMRT) at (P ≤ 0.05) between treatments.
Results and Discussion
Plant growth parameter
Up to 2 weeks after drought treatment, control plants and plants that had been treated with drought stress were not significantly different for leaf number, leaf area and fresh weight of Chinese cabbage. At week 3 after drought treatment, drought stress treated plants were significantly reduced leaf number, leaf area, and fresh weight of Chinese cabbage subjected to drought stress when compared with those of the control plants (Table 1). Compared with those of the control plants, at three week after drought stress, the leaf area and fresh weight of plants subjected to drought stress decreased by 21.7% and 25.0%, respectively. A similar reduction in leaf area (25%) and fresh weight (34%) has been reported in springtime-growing Chinese cabbage (cv. Chunkwang) subjected to drought stress (Shawon et al., 2017). Other studies have also showed that a significant reduction in leaf number, leaf area, and fresh weight in several Brassica species including Brassica rapa (Issarakraisila et al., 2007), Brassica oleracea (Wu et al., 2012), Brassica napus (Qaderi et al., 2006; Naderikharaji et al., 2008; Sangtarash et al., 2009), subjected to water stress. Water deficiency reduces plant growth by reducing turgor pressure, cell expansion, cell wall synthesis, and cell division (Hsiao, 1973; Jaleel et al., 2009, Anjum et al., 2011). Under water stress condition, plants try to adapt by reducing their leaf area for limited transpiration (Mahajan and Tuteja, 2005; Manivannan et al., 2007; Anjum et al., 2011). These results suggest that drought intensity, drought period, growing temperature and seedling age significantly influence plant growth parameters of Chinese cabbage under drought stress condition.
Table 1. Effect of drought stress on leaf number, leaf area and fresh weight of Chinese cabbage
yMeans within columns at each week sharing the same letter are not significantly different by Duncan’s Multiple Range Test at P≤0.05 (n = 5).
Free amino acid
In the present study, the content of aspartate, glutamate, serine, glutamine, threonine, arginine, norvaline, and tryptophan together accounted for approximately 80% of the total free amino acid pool in both the control and drought-stressed plants (Table 2, Fig. 3). At week 2 of treatment, there was a reduction in free amino acid content in plants subjected to drought stress which might be responsible for the reduced level of free amino acids (including aspartate, glutamate, GABA, valine, and norvaline) (Table 2). However, two weeks of drought-stress increased serine and glutamine content in Chinese cabbage when compared with those in the control. Furthermore, after two weeks of drought treatment, vitamin-U and lysine were not detected in drought-stressed plants, whereas, these were detected in untreated plants. For up to two weeks of treatment, the content of total free amino acids was significantly reduced in the drought-stressed Chinese cabbage than in the untreated control (Table 2). Under stress condition, a change in free amino acid content in plants is one of the earliest symptoms (Rai, 2002). Generally, it has been reported that amino acid accumulation increases in plants such as sunflower and sorghum, under drought stress because of osmotic adjustment to alleviate detrimental effects (Morgan, 1984; Yadav et al., 2005; Manivannan et al., 2007). The accumulation of amino acids during drought stress might be due to protein hydrolysis (Farooq et al., 2009). In contrast, drought stress decreased total free amino acid content in some plant species. For instance, El-bassiouny and Bekheta (2005) reported that water deficiency reduced total free amino acid content in wheat (Triticum aestivum). They showed that water deficiency decreased aspartate, valine, and lysine contents in wheat, whereas, there was an increase in glutamine and serine content. Glutamine accumulation has also been reported to increase in the leaves of rice (Oryza sativa) subjected to water deficiency (Yang et al., 2000). The content of aspartate and glutamate decreased in 28-d-old Lotus corniculatus, but the content of serine and glutamine increased under drought stress (Diaz et al., 2005). Glutamate act as a nitrogen source in the synthesis of glutamine from ammonia, and its synthesis is influenced by water stress condition in plants (Liaw and Eisenberg, 1994). During water stress, the activation of saccharopine pathway is one of the reasons for the decrease in aspartate content, leading to the accumulation of glutamine (Michaletti et al., 2017). The increase in serine content in plants might be due to high rate of photorespiration under water stress condition (Diaz et al., 2005). Additionally, age, cultivar, and drought intensity might be other reasons for the decreasing trend in total amino acid accumulation in Chinese cabbage under drought stress. Hausler et al. (2014) reported that amino acid catabolism depends on plant species and their developmental stages.
Table 2. Effect of drought stress on individual free-amino acid content in Chinese cabbage
yND = not detected.
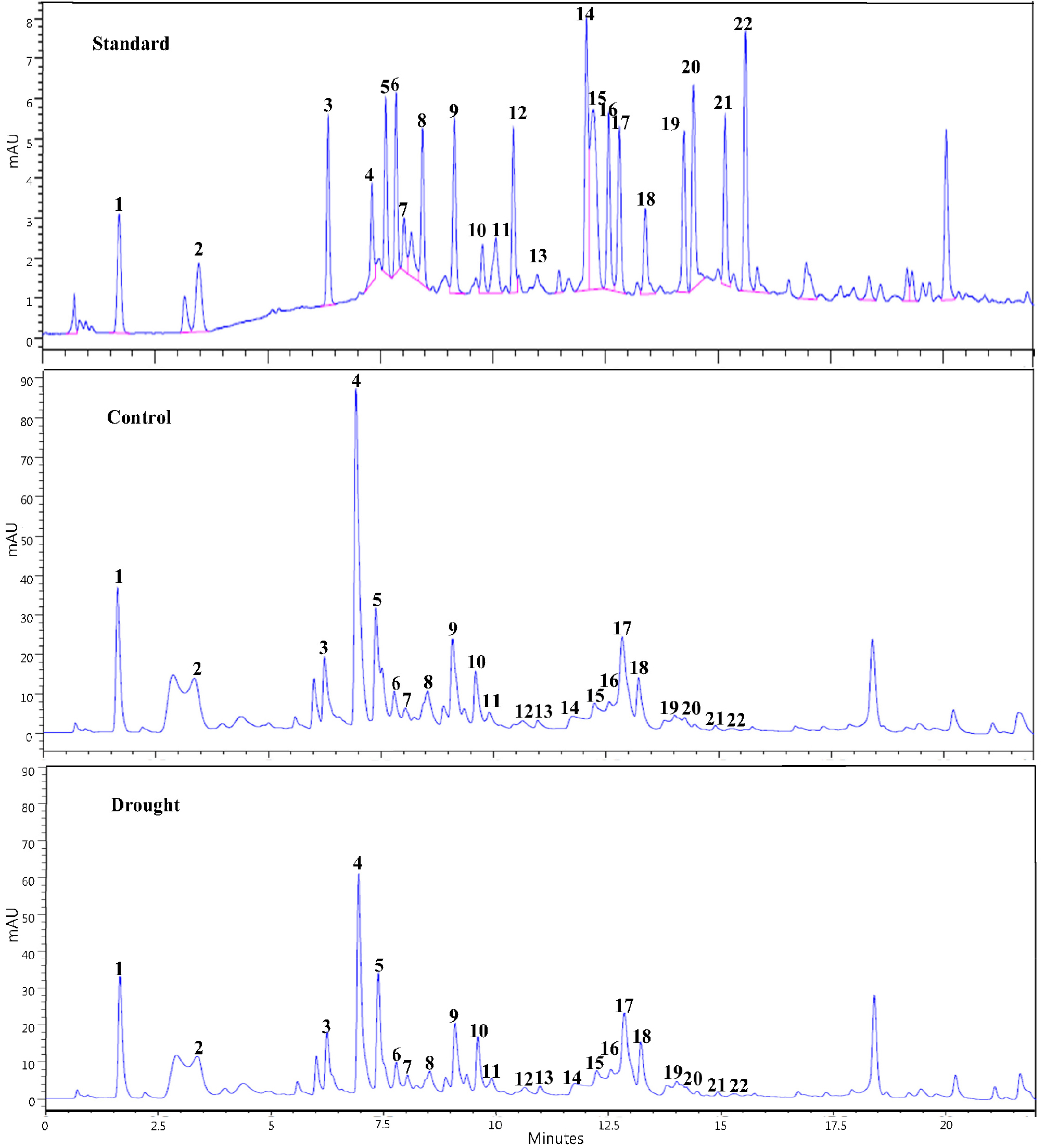
Fig. 3.
High-performance liquid chromatography (HPLC) of total free amino acid in Chinese cabbage at 1 week after drought stress. The soil water content in control plant and drought-treated plant was maintained at 10% and 30%, respectively. 1, Aspartate; 2, Glutamate; 3, Asparagine; 4, Serine; 5, Vitamin U; 6, Glutamine; 7, Histidine; 8, Glycine; 9, Threonine; 10, Arginine; 11, Alanine; 12, GABA; 13, Tyrosine; 14, Cystine; 15, Valine; 16, Methionine; 17, Norvaline; 18, Tryptophan; 19, Phenylalanine; 20, Isoleucine; 21, Leucine; 22, Lysine.
Carotenoid content
For up to two weeks of treatment, lutein, zeaxanthin, α-carotene, β-carotene, and total carotenoid were identified, and their content was not significantly different between the control and drought-stressed plants (Table 3, Fig. 4). At three weeks of treatment, the content of α-carotene and β-carotene in drought-stressed plants were significantly higher than that in the untreated plants (Table 3). In general, the carotenoid content was not affected or marginally reduced in drought-stressed plants. Zushi and Matsuzoe (1998) reported that water deficiency had no effect on the content of xanthophyll and β-carotene in tomatoes (Lycopersicon esculentum). In canola (Brassica napus), the carotenoid content was not significantly different between the control and drought-stressed plants under ambient UVB (Sangtarash et al., 2009).
Table 3. Effect of drought stress on total carotenoid content in Chinese cabbage
yMeans within columns at each week sharing the same letter are not significantly different by Duncan’s Multiple Range Test at P≤0.05 (n = 5).
A significant decrease in carotenoid content has been reported in several crops, subjected drought stress (Haisel et al., 2006). Drought stress might be negatively associated with photosynthesis process, which could be hindrance in the formation of photosynthetic pigments, including carotenoid (Zeid and Sheedeed, 2006). Sairam and Saxena (2000) reported that the antioxidant and pigment responses of plants are correlated during water stress. Preliminary studies have also showed that the antioxidants APX and SOD are unaffected in springtime-growing cultivar of Chinese cabbage subjected to three weeks drought stress (Shawon et al., 2017).
Carotenoid biosynthesis depends on the growing stage of plants (Cazzonelli and Pogson, 2010). Carotenoid content showed a decreasing trend with age in both drought-tolerant and susceptible cultivars of wheat (Sairam and Saxena, 2000). Carotenoid content in tobacco (Nicotiana tabacum) leaves also decreased with age (Prochazkova et al., 2009). The decrease in carotenoid content in older leaf correlated with leaf senescence, declining the content of photosynthetic pigments because of the violaxanthin cycle (Prochazkova et al., 2009).
In contrast, at three weeks after drought treatment, the total carotenoid content significantly increased in drought-stressed Chinese cabbage than in the untreated control (Table 3). Increased level of individual carotenoids, including α-carotene and β-carotene might have increased the total carotenoid content in three week drought-stressed plants. It is assumed that the intensity of drought and production of β-carotene are positively correlated. Simkin et al. (2008) and Yun et al. (2018) reported that drought intensity leads to the formation of ROS in plants. It is necessary for organisms to suppress the production of ROS (Jang and Park, 2018). However, β-carotenoid helps reduce the production of singlet oxygen in photosynthetic tissue by quenching triplet chlorophyll (Farooq et al., 2009).
Proline content
In the present study, the content of proline did not significantly differ between drought-treated and control Chinese cabbage (Figs. 5 and 6). Similar results have also been reported by other studies. Pirzad et al. (2011) showed that proline content in Matricaria chamomilla L. was not affected by water deficiency, when field capacity maintained at 55%-100%. Chutia et al. (2012) reported that drought stress (osmotic potential of 0.15 bar by PEG 6000) did not significantly affect the proline content of four different rice cultivars.
Proline is an organic osmolyte that helps in osmotic adjustment in plants under stress, such as high salinity, high temperature, and drought stress (Ueda et al., 2001; Ashraf and Foolad, 2007; Jaleel et al., 2009). A significant increase in proline accumulation in plants is a common phenomenon under drought stress condition (Choudhary et al., 2005). Hayat et al. (2008) reported that during drought stress, proline accumulation significantly increased in 20-d and 30-d old tomato seedlings. Turkan et al. (2005) showed that in 35-d-old bean cultivar seedlings (P. vulgaris, and P. acutifolius) subjected to drought stress (PEG 6000) for two weeks, there was an increase in proline accumulation. Furthermore, drought stress-induced, increase in proline synthesis was observed in chick pea (Mafakheri et al., 2010) and wheat (Vendruscolo et al., 2007). Reduced glutamate content might be responsible for the non-significant proline accumulation in Chinese cabbage observed in the present study, as glutamate is the major source of proline synthesis in plants under osmotic stress (Choudhary et al., 2005).
In conclusion, the result of the present study showed that drought stress in Chinese cabbage significantly reduced plant growth parameters and affected the content of total and individual free amino acids and carotenoid. However, during three weeks of drought stress, there was no significant change in the content of proline in Chinese cabbage.