Introduction
Materials and Methods
Chemicals and reagents
Plant material
Cell culture
MTT assay
Measurement of melanin content
Intercellular tyrosinase activity
Western blot analysis
Zebrafish Maintenance
Effects on zebrafish embryo pigmentation
Data analysis
Results
Cytotoxicity of RCB extract in B16F10 mouse melanoma cells
RCB extract inhibits α-MSH-induced melanin synthesis and intercellular tyrosinase activity in B16F10 melanoma cells
RCB extract inhibits α-MSH-induced melanogenesis- related protein expression
RCB extract inhibits melanogenesis through inhibition of the ERK pathway
RCB extract inhibits α-MSH-induced melanogenesis in zebrafish
Discussion
Introduction
In mammals, melanin is the major pigment responsible for the color of human skin and hair. It is synthesized in the melanosomes of melanocytes (Solano, 2014). This process, called melanogenesis, is a complex phenomenon that occurs in melanocytes by the action of melanogen, cytokines, and melanogenic enzymes, such as tyrosinase, tyrosinase-related protein 1 (TRP-1), and tyrosinase-related protein 2 (TRP-2) (Sugumaran, 2016; Videira et al., 2013). Alpha-melanocyte- stimulating hormone (α-MSH) binds to its specific receptor (MC1R), resulting in the activation of the GTP-binding protein, which in turn stimulates adenylate cyclase to produce cyclic adenosine monophosphate (cAMP). cAMP increases the expression of microphthalmia-associated transcription factor (MITF), which in turn stimulates tyrosinase gene expression. Importantly, tyrosinase catalyzes the rate limiting reaction of melanogenesis and thus, melanin production is mainly controlled by the expression and activity of tyrosinase (Busca and Ballotti, 2000; Hyun et al., 2021; Levy et al., 2006; Ohguchi et al., 2006; Shin et al., 2010).
Mitogen-activated protein kinases (MAPKs) also influence melanogenesis. Inhibition of the extracellular signal-regulated kinase (ERK) pathway increases tyrosinase activity in B16 melanoma cells, whereas sustained ERK activation reduces melanin synthesis via MITF degradation and subsequent downregulation of tyrosinase and TRP-1 (Hwang et al., 2016; Park et al., 2020). Surprisingly, the activation of the p38 MAPK pathway has been reported to increase melanin synthesis by stimulating MITF expression and tyrosinase transcription. This illustrates the complexity of the involvement of MAPK in melanogenesis (Sun et al., 2020).
The zebrafish embryo is a popular vertebrate model system for biochemical research with high physiological and genetic similarities to mammals along with the advantage that it develops fast, lays 100 - 200 eggs at a time, and the eggs are transparent, making it easy to visualize the experiment (MacRae and Peterson, 2015; Rannekamp and Peterson, 2015). Therefore, zebrafish embryo screening has emerged as an alternative approach to animal experiments. Moreover, zebrafish embryos were frequently used in the early search for whitening agents due to the fact that the body is transparent, making it easy to observe the production of pigments.
Melanin pigments can be observed on the zebrafish surface, allowing simple observation of the pigmentation process without complicated experimental procedures (Jinpeng et al., 2020). Several studies have focused on the inhibition of melanogenesis and the prevention of abnormal pigmentation for medical and cosmetic benefits (Solano et al., 2006). Strategies for screening melanogenesis inhibitors include in vitro and in vivo approaches (Gunia-Krzyżak et al., 2016). Numerous melanogenesis inhibitors are currently being utilized as pharmaceutical or cosmetic additives; however, owing to their adverse side effects (skin irritation, cytotoxicity), low formulated stability, and poor skin penetration, their use is still limited (Hermanns et al., 2020). Due to these safety concerns, the identification and isolation of active compounds from natural sources that prevent pigment disturbances have attracted increasing interest.
Ranunculus chinensis Bunge (RCB) is a perennial plant that belongs to buttercups. According to the dictionary of Chinese medicine, it is reported that it is effective in reducing inflammation, swelling, rheumatoid arthritis, and skin diseases, and eliminating parasites. It is used to treat hepatitis, cirrhosis, and schizophrenia (Nigel and Feng, 1998). However, the pharmacological effects of RCB have not yet been proven. Therefore, in this study, we verified the whitening effects of RCB on α-MSH-induced B16F10 melanoma cells.
Materials and Methods
Chemicals and reagents
L-DOPA, 3-(4, 5-dimethylthiazol-2-yl)-2, 5-diphenyltetrazolium bromide (MTT), phenylthiourea (PTU), and α-MSH were purchased from Sigma-Aldrich (St. Louis, MO, USA). Antibodies specific for phospho-ERK1/2, total ERK1/2, and the inhibitor PD98059 were purchased from Cell Signaling Technology (Beverly, MA, USA). Antibodies against tyrosinase, TRP-1, TRP-2, and MITF were obtained from Abcam (Cambridge, UK).
Plant material
Ranunculus chinensis Bunge (RCB) were collected from Mungyeong, Gyeongsangbuk-do. The materials for extraction were freeze-dried and ground into a fine powder using a blender. The dried powder (50 g) was extracted with 70% EtOH extract (70% EtOH, 1L) at room temperature for 2 d. The yield of 70% EtOH extract was 11.5%.
Cell culture
B16F10 murine melanoma cell line was purchased from the Korean Cell Line Bank (Seoul, South Korea). These cells were maintained at subconfluence in a 95% air and 5% CO2 humidified atmosphere at 37℃. The medium used for the routine subculture was Dulbecco’s modified Eagle’s medium (DMEM) containing 10% fetal bovine serum (FBS), penicillin (100 units/mL), and streptomycin (100 μg/mL).
MTT assay
Cell viability was determined using MTT assay. B16F10 cells (5.0 X 104) were cultured in 24-well plates for 18 h, followed by treatment with various concentrations (25, 50, and 100 μg/mL) of RCB extract for 48 h. Briefly, MTT was added to cells and the formazan crystals were dissolved in dimethyl sulfoxide (DMSO). Absorbance was measured at a wavelength of 540 nm. The percentage of cells showing cytotoxicity was determined relative to that of the control group.
Measurement of melanin content
The amount of melanin in B16F10 cells was measured according to a previously published method with slight modifications (Hosoi et al., 1985). The cells were treated with RCB extracts and α-MSH for 48 h at 37℃. After media removal, cells were washed with cold phosphate-buffered saline (PBS) and cell pellets were dissolved in 1 N NaOH for 1 h at 80℃. Spectrophotometric analysis of melanin content was performed at an absorbance of 405 nm. Each experiment was performed in triplicates.
Intercellular tyrosinase activity
Intercellular tyrosinase inhibitory activity was determined using a previously described method, with slight modifications (Busca et al., 1996). Briefly, B16F10 cells (1.0 × 105) were seeded in 60 ㎜ dishes and the plates were then incubated at 37℃ in a humidified atmosphere with 5% CO2 for 3 d. Next, seeded B16F10 cells were lysed with phosphate buffer containing 1% Triton X-100. Lysates were clarified by centrifugation for 15 min at 13,000 rpm. After the quantification of protein levels and concentration adjustment using lysis buffer, each lysate containing identical amounts of protein along with the test samples were added to 96-well plates, and 15 mM L-DOPA was added to each well. After incubation at 37℃, we measured absorbance at 475 nm using an ELISA reader.
Western blot analysis
After α-MSH-stimulated B16F10 cells were incubated for 48 h, they were washed twice with cold phosphate-buffered saline (PBS). The cells were allowed to lyse in lysis buffer (RIPA buffer, 1% NP-40, 1% protease inhibitor cocktail) for 1 h, collected in microtubes, and then centrifuged at 15,000 rpm for 15 min at 4℃. The supernatants were transferred to new microtubes. The protein content of the cell lysates was determined using Bradford reagent (Bio-Rad, CA, USA) with bovine serum albumin (BSA) as a standard. After heating at 70℃ for 10 min, equal amounts of the cell lysates were separated by 4 - 12% Bis-Tris mini gel electrophoresis (Invitrogen, MA, USA) and transferred to a nitrocellulose membrane (Invitrogen). The membrane was then washed with Tris- buffered saline (TBS; 20 mM Tris base, 137 mM NaCl, pH 7.6) containing 0.1% Tween 20 (TTBS) and blocked with TTBS containing 5% skim milk solution for 2 h. The membrane was incubated overnight at 4℃ with the primary antibodies diluted in TTBS (1:1000). The primary antibodies (TRP-1, TRP-2, tyrosinase, and MITF) were purchased from Abcam. The membranes were washed four times with TTBS. Each membrane was incubated for 1 h with secondary peroxidase-conjugated goat immunoglobulin G (IgG; 1:5000) and washed four times with TTBS. The target proteins were detected using an enhanced chemiluminescence (ECL) solution. Immunoreactive bands were detected and exposed to X-ray films. For quantitative analysis, the amount of protein expression was determined by the Image lab software version 5.2.1 (Bio-Rad).
Zebrafish Maintenance
Mature zebrafish were obtained from a commercial dealer and kept in a water tank with a closed circulation filtration system, maintaining a water temperature of 28.5℃, with a 14/10 h light/dark cycle (Yuma et al., 2019). They were fed live brine shrimp (San Francisco Bay Brand, Inc., Newark, CA, USA) thrice a day. Embryos were obtained from natural spawning that was induced in the morning by turning the light on. Embryo collection was completed within 30 min. The collected embryos were placed in zerbrafish embryo medium, reared for 24 h, and treated with various concentrations of RCB extract.
Effects on zebrafish embryo pigmentation
Mature male and female zebrafish were placed in an egg collection tank the day before egg collection, and the eggs were collected the day after 1 - 2 h of the light cycle period. The collected eggs were placed in a zebrafish embryo medium and incubated for 24 h, and each sample was treated by varying concentrations of RCB extract. Since the degree of sample permeation of the chorion was not known at the time of sample treatment, a hole was made in the chorion of each egg during treatment, and the eggs that showed toxicity in the sample 24 h after the sample treatment were removed. The second sample was treated after the complete removal of the chorion of the remaining eggs. After 48 h of treatment, the degree of pigment development in the sample treatment sphere compared with that in the control group was observed under a substantive microscope.
Data analysis
All data are expressed as mean ± S.D (n=3). Significant differences between the tested groups were determined using unpaired Student’s t-test, and statistical significance was set at p < 0.05.
Results
Cytotoxicity of RCB extract in B16F10 mouse melanoma cells
To investigate whether RCB extract has cytotoxic effects, we first performed MTT assays to determine whether RCB extract was cytotoxic to B16F10 cells. Cell viability was measured 48 h after treatment with 25, 50, and 100 μg/mL RCB extract. We observed that RCB extract had no significant effect on the cell viability (Fig. 1). Thus, a dose of 25 - 100 μg/mL was selected to determine the effects of RCB extract on melanogenesis and tyrosinase activity.
RCB extract inhibits α-MSH-induced melanin synthesis and intercellular tyrosinase activity in B16F10 melanoma cells
Next, we investigated the effects of RCB extract on α- MSH-induced melanogenesis. In this study, α-MSH (100 nM) was used to stimulate melanogenesis in B16F10 cells. Cells (2.0 × 104) were pre-incubated for 18 h and melanin content was determined after incubation of B16F10 cells treated with α-MSH (100 nM) and RCB extract for 72 h at 37℃ in a 5% CO2 atmosphere by analyzing the amount of melanin secreted into the medium. Cells treated with α-MSH alone were able to markedly increase melanin content compared with normal cells. RCB extract inhibited α-MSH-induced melanin synthesis in a dose-dependent manner (Fig. 2A).
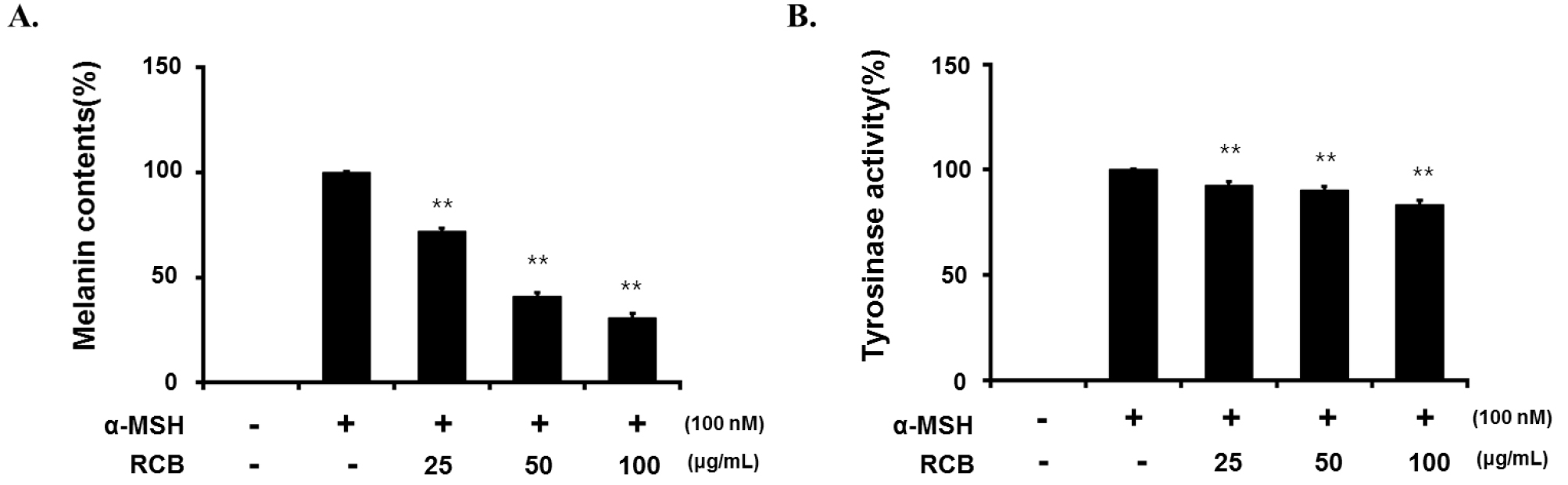
Fig. 2.
Inhibitory effect of RCB extract on melanogenesis and intracellular tyrosinase activity in B16F10 cells. B16F10 cells (5.0 × 104 cells/ml) were pre-incubated for 18 h in 24 well plate and melanin content was determined after incubation of B16F10 cells treated with α-MSH (100 nM) and RCB extract (25, 50, and 100 μg/mL) for 72 h at 37℃ in a 5% CO2 atmosphere. The melanin content (A) and cellular tyrosinase activity (B). The data represent mean ± S.D of triplicate experiments. *p < 0.05 and **p < 0.01.
Tyrosinase is a key enzyme in melanin biosynthesis, inhibition of which is a major strategy for the development of new whitening agents (Vontzalidou et al., 2012). Therefore, we investigated the effects of RCB extract treatment on intercellular tyrosinase expression. RCB extract significantly decreased α-MSH-induced intercellular tyrosinase activity in a dose-dependent manner (Fig. 2B).
RCB extract inhibits α-MSH-induced melanogenesis- related protein expression
We examined the influence of RCB extract on the expression of the melanogenesis-related proteins tyrosinase, TRP-1, TRP-2, and MITF by western blotting using cell lysates treated with different concentrations of RCB extract for 72 h.
As shown in Fig. 3, RCB extract at 100 μg/mL suppressed protein expression of TRP-1, TRP-2, and tyrosinase in comparison with the α-MSH treated group. MITF is a key transcription factor that regulates the expression of most melanogenesis-related genes (Qiyun et al., 2018). To understand the transcriptional regulation of TRP-1, TRP-2, and tyrosinase, we investigated the effect of RCB extract on MITF expression. The RCB extract effectively suppressed the expression of MTIF at a concentration of 100 μg/mL, although not in a concentration-dependent manner on the expression of MITF. This requires the need to study more detailed melanin synthesis cell signaling through additional various studies. It has been suggested that the downregulation of melanogenesis- related inhibition of melanin synthesis by RCB extract proteins could be associated with MITF signaling.
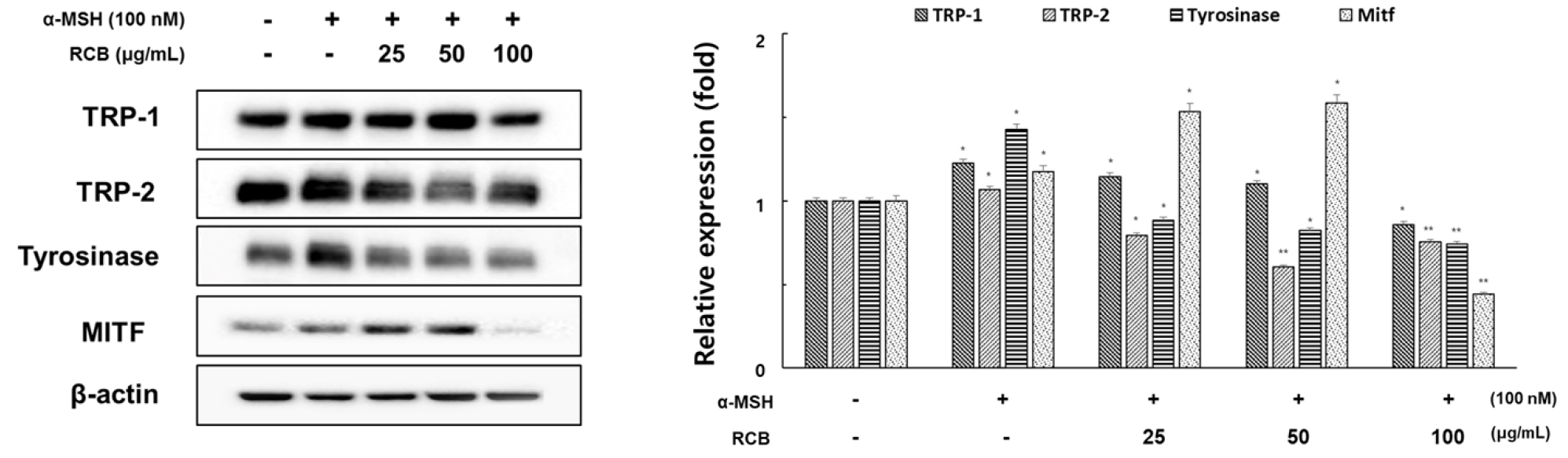
Fig. 3.
Inhibitory effects of RCB extract on the protein level of tyrosinase, TRP-1, TRP-2, and MITF in α-MSH-stimulated B16F10 cells. B16F10 cells (1.0 × 105 cells/ml) were pre-incubated for 18 h in 6 well plate and then stimulated with α-MSH (100 nM) and RCB extract (25, 50, and 100 μg/mL) for 24 h. The expression levels of tyrosinase, TRP-1, TRP-2 and MITF were examined by western blot analysis. Equal protein loadings were confirmed by tyrosinase, TRP-1, TRP-2, MITF and β‑actin expression. Protein levels were quantified using image lab software version 5.2.1. The data represent mean ± S.D of triplicate experiments. *p < 0.05 and **p < 0.01. (vs. the α-MSH-treated group).
RCB extract inhibits melanogenesis through inhibition of the ERK pathway
To understand how RCB extract regulates MITF expression, we investigated the time-dependent effects of RCB extract treatment on ERK phosphorylation. The levels of ERK protein expression were determined according to α-MSH treatment time (10 min - 72 h). Phosphorylation of ERK protein was most activated 15 min after α-MSH treatment. (Fig. 4A). Under the same conditions, RCB extract treatment increased ERK phosphorylation. Phosphorylation of ERK induced by RCB extract was inhibited by PD98059, a selective inhibitor of the ERK pathway (Fig. 4B).
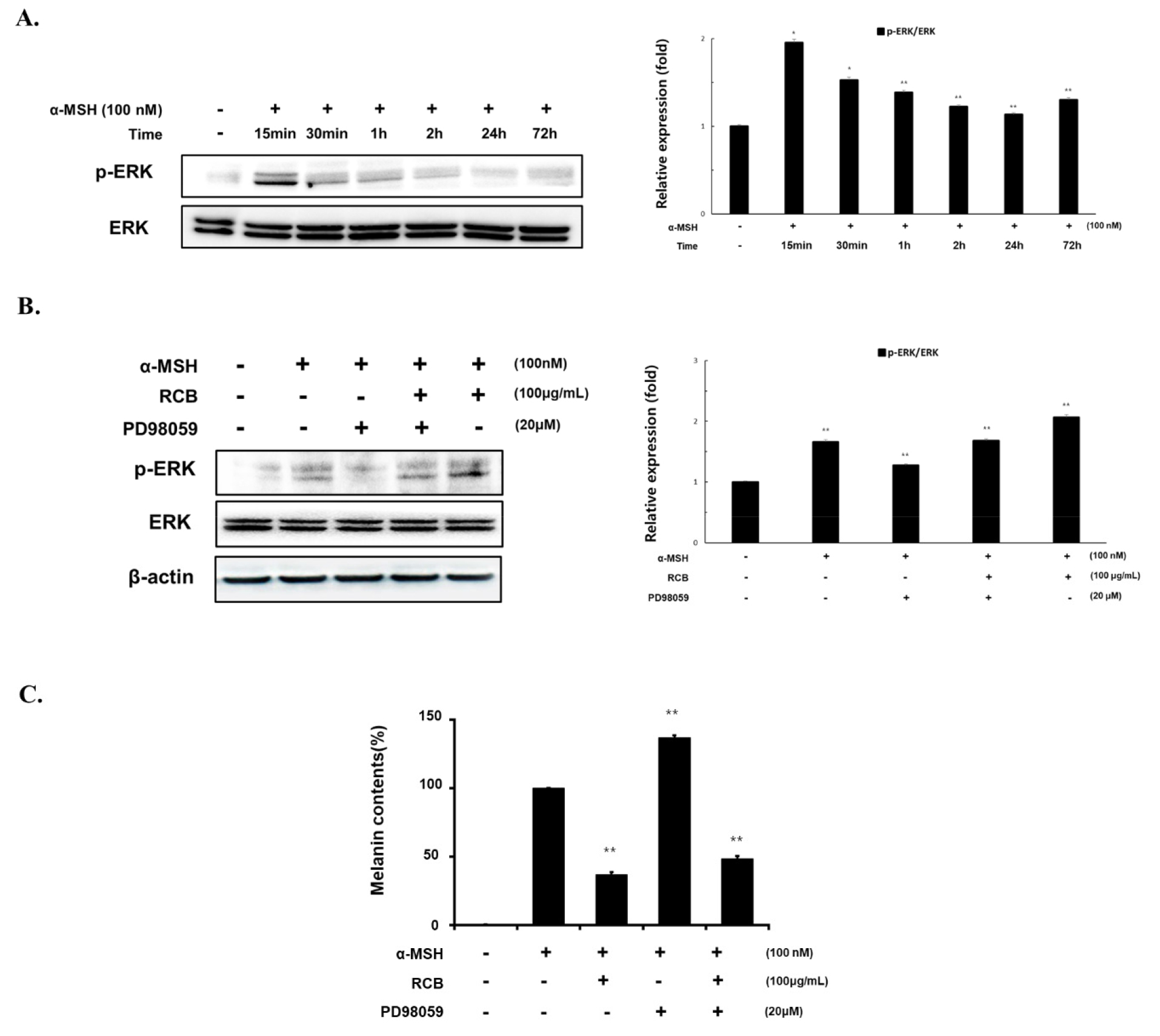
Fig. 4.
Effects of RCB extract on ERK signaling pathway in α-MSH-stimulated B16F10 cells. (A) B16F10 cells (1.5 × 105 cells/ml) were treated with α-MSH (100 nM) for the indicated time points. The expression levels of ERK were examined by western blot analysis. (B) B16F10 cells (1.5 × 105 cells/ml) were treated with α-MSH alone or α-MSH and RCB extract (100 μg/mL) in the absence or presence of PD98059 (20 μM). Changes in phosphorylated ERK expressions were examined 15 min after treatment. The expression levels of ERK were examined by western blot analysis. Protein levels were quantified using image lab software version 5.2.1. (C) B16F10 cells (5.0 × 104 cells/ml) were pre-incubated for 18 h in 24 well plate and then stimulated with α-MSH (100 nM) and RCB extract (25, 50, and 100 μg/mL) in the absence or presence of PD98059 (20 μM). Melanin contents measured at 48 h after treatment. The data represent the mean ± S.D of triplicate experiments. *p < 0.05 and **p < 0.01.
To further investigate the effect of RCB extract induced ERK phosphorylation on melanin production, melanin content was evaluated in RCB extract treated cells in the presence of PD98059. The extent of melanin inhibition by RCB extract was increased by PD98059 treatment (Fig. 4C). These results indicate that RCB extract inhibits melanogenesis by activating ERK signaling, followed by downregulation of melanogenic proteins.
RCB extract inhibits α-MSH-induced melanogenesis in zebrafish
Zebrafish have melanin pigments on their surface, allowing simple observation of the pigmentation process without complicated experimental procedures (Chang et al., 2015). Therefore, we tested the effects of RCB extract on zebrafish pigmentation. We used PTU, a sulfur-containing tyrosinase inhibitor that is widely used in zebrafish research, as a positive control (Lee et al., 2017). As shown in Fig. 5, RCB extract treated embryos showed a remarkable inhibition of body pigmentation in a concentration-dependent manner without toxicity during the experimental period.
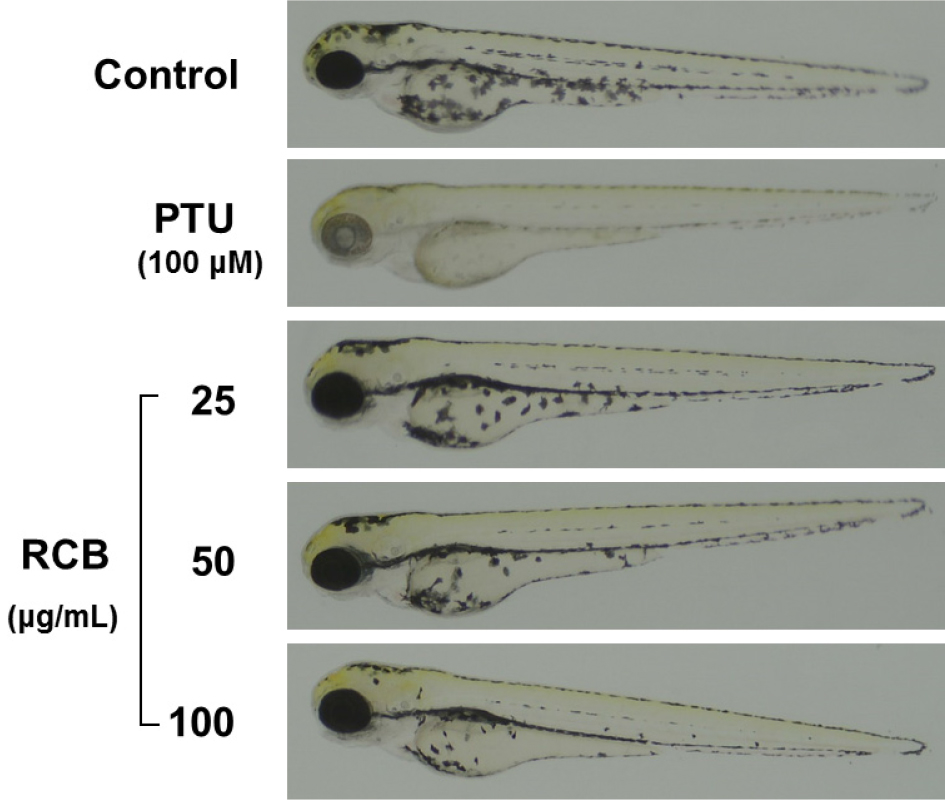
Fig. 5.
Inhibitory effects of RCB extract on melanogenesis in zebrafish embryo. Representative photographs of zebrafish. Synchronized embryos were treated with melanogenic inhibitors at the indicated concentrations. RCB extracts were dissolved in 0.1% DMSO and then added to the embryo medium. The effects on the pigmentation of zebrafish embryo were observed under the stereomicroscope.
Discussion
Medicinal plants are used as medicines for the treatment and prevention of diseases, and have been widely utilized as home medicines and food additives (Ivanova et al., 2005). The search for and characterization of new pharmaceutical and healthcare products from natural sources has increased in recent years. In particular, as the cosmetic industry places importance on function, interest in natural cosmetics using safe natural ingredients is increasing. Such products may be beneficial to humans as antioxidants, anti-inflammatory drugs, and antihypertensive drugs (Whitney and Pugliese, 2014). Plant-derived products are increasingly being accepted and adopted by the cosmetics industry. Consequently, there is considerable research interest in the use of natural products, such as chemical extracts from medicinal plants, herbs, and spices, for the development of alternative food additives and cosmetic products (Yaar et al., 2006). Therefore, in this study, the skin-whitening activity of the medicinal plant RCB extract was investigated.
First, to exclude cytotoxicity in B16F10 cells, we used the MTT assay to investigate the effects of RCB extract on the viability of B16F10 cells. Cells retained almost the same viability when exposed to RCB extract at concentrations less than 100 μg/mL for 48 h. However, it showed cytotoxicity at concentrations above 100 μg/mL. This means that the series of effects that RCB extract exhibits on cells are unique effects that have nothing to do with changes in the survival rate of cells.
Melanin levels are directly correlated with the protein levels and activity of tyrosinase (Shibahara et al., 2000). We investigated whether RCB extract inhibits melanin synthesis in the presence of α-MSH and observed that RCB extract inhibited melanin synthesis and tyrosinase activity in a concentration-dependent manner without cytotoxicity (25, 50, and 100 μg/mL) in B16F10 cells. These results demonstrated that RCB extract inhibited melanin synthesis and downregulated tyrosinase activity in α-MSH-stimulated B16F10 cells.
MITF is a transcription factor in several cell types and is involved in the regulation of melanocyte differentiation, pigmentation, proliferation, and survival (Levy et al., 2006). Specifically, MITF is a major transcriptional regulator of melanogenic enzymes such as tyrosinase. At higher levels, α-MSH binds to its receptor, increasing cAMP levels. It can then activate cAMP-dependent protein kinase (PKA), which is usually used to induce the phosphorylation of cAMP response element-binding protein (CREB) and increase MITF protein levels. MITF induces the expression of tyrosinase and other melanogenic enzymes, which ultimately increases melanin synthesis (Mas et al., 2003). To determine whether the suppressive activity of RCB extract is associated with the expression levels of melanogenesis-related proteins, such as MITF, tyrosinase, TRP-1, and TRP-2, cells were exposed to α-MSH with or without RCB extract treatment, and protein extracts were then subjected to western blot analysis. RCB at 100 μg/mL suppressed protein expression of TRP-1, TRP-2, and tyrosinase by 29.8%, 24.4%, and 48%, respectively, in comparison with the control group. Moreover, RCB extract did not inhibit MITF protein expression in a concentration- dependent manner but significantly suppressed MITF expression at 100 μg/mL by 62.3%. It has been suggested that the downregulation of melanogenesis-related proteins by RCB extract is associated with MITF signaling. However, considering natural mechanisms that can downregulate melanin formation, the ERK signaling pathway has been reported to be involved in the phosphorylation of MITF as a result of ERK activation and subsequent promotion of MITF degradation (Price et al., 1998). Therefore, we examined the influence of RCB extract treatment on ERK activation to further understand the molecular mechanisms involved in RCB extract pigmentation.
Our results showed that RCB extract significantly enhanced ERK phosphorylation. To further determine whether RCB extract suppressed melanin synthesis through the ERK pathway, we treated B16F10 cells with the ERK-specific inhibitor PD98059. We found that the suppressive effects of RCB extract on melanin synthesis were partially diminished by prior inhibition of the ERK pathway. This finding suggests that RCB extract induces anti-melanogenesis in B16F10 cells and is involved in the ERK-mediated pathway.
We further validated the anti-melanogenic activity of RCB extract in an in vivo zebrafish model. Zebrafish are useful vertebrate model systems to investigate the depigmentation activity of melanogenic regulatory compounds (Choi et al., 2007). In this study, zebrafish embryos were treated with RCB extract in a dose-dependent manner without any oral toxicity during the experimental period. RCB extract treated embryos showed a reduction in body pigmentation in a concentration-dependent manner compared with the untreated control. These results demonstrate that RCB extract inhibits melanogenesis in vivo by regulating the transcription factor MITF and its targets TRP-1, TRP-2, and tyrosinase.
In summary, we found that RCB extract inhibits melanogenesis in α-MSH-treated B16F10 cells without any cytotoxicity. RCB extract induced ERK phosphorylation, which led to downregulation of MITF and tyrosinase expression. Furthermore, RCB extract inhibits melanogenesis in a zebrafish model. These results suggest that RCB extract can be used to treat various hyperpigmentation disorders, as well as in whitening cosmetics. Further studies on the separation and identification of the active ingredients of RCB extract are needed.