Introduction
Materials and Methods
Plant materials and cultivation
Morphological traits
Anthracnose inoculum preparation and inoculation
Disease index
Statistical analysis
Results and Discussion
Morphological traits
Distribution of disease index
Correlation between morphological traits and disease index
Principal component analysis (PCA)
Introduction
Watermelon (Citrullus lanatus) is an important crop with a production of 100 million tons in 2022 worldwide, and 489,897 tons in Korea (FAO, 2023). In modern agriculture, plant diseases are one of the important factors which reduce fruit quality and yield. Colletotrichum orbiculare is a fungal pathogen that causes anthracnose in watermelon and other cucurbits such as cucumber, melon, squash and pumpkin (Wasilwa et al., 1993). C. orbiculare cause symptoms all the above-ground parts of plants, such as leaf, stem and fruits. C. orbiculare causes dark brown lesion on leaf and as the disease progresses, the center of the lesion is pierced. In fruits, symptoms start as a small black spot, lesions sink and show spores on the lesion (Anderson and Walker, 1962). This anthracnose can therefore reduce fruit quality and cause loss in fruit yield.
Pathological races of C. orbiculare were defined as race 1-3 based on watermelon, cucumber and squash (Goode, 1958). Race 4 (Dutta et al., 1960) and 5, 6, and 7 (Jenkins et al., 1964) were defined later. In watermelon, race 1, 2 and 3 were identified by pathogenicity on ‘Charleston Gray’, ‘Congo’, ‘Fairfax’, ‘Garrison’ and ‘New Hampshire Midget’ (Goode and Winstead, 1957). ‘Garrison’ and ‘New Hampshire Midget’ are susceptible to all races, ‘Charleston Gray’, ‘Congo’, and ‘Fairfax’ are resistant to race 1 and 3, but susceptible to race 2. Wasilwa et al. (1993) examined the pathogenicity of 92 C. orbiculare isolates from the U.S and segregated them into two races. Matsuo et al. (2022) reported races of anthracnose isolates distributed in Japan. But little is known about distribution of races with in Korea (Huh et al., 2010a).
Chemical control, field management, and crop rotation have been used to control anthracnose (Patel et al., 2023). Effectiveness of fungicides have been evaluated in the past (Byrd-Masters, 2018; Egel and Marchino, 2018; Keinath, 2018). Potential of endophytic fungi from watermelon leaf for biocontrol has been evaluated previously (Pradeep et al., 2022).
Screening for resistant and development of resistant cultivars have been conducted since 1937. Layton (1937) identified five resistant cultivars from Africa and carried out breeding of resistant commercial cultivars. The first resistant commercial cultivars being released in 1949 (Andrus, 1955). After the pathological races of C. orbiculare were defined, Winstead et al. (1959) examined the resistance of 86 watermelon cultivars to race 1 and 3, and reported 10 resistant cultivars as well as the resistance gene to anthracnose race 1 and 3, namely, Ar-1. The SNP marker CL 14-27-9 was designed as the diagnostic marker of race 1 resistance (Jang et al., 2019) and used for developing resistant cultivar. Commercial cultivars resistant to race 1 were developed by companies in Japan, Switzerland and the USA (Patel et al., 2023), however, many cultivars are found to be susceptible to race 2 (Keinath, 2017). Previously, watermelon cultivars resistant to anthracnose race 3 were developed in Korea (Huh et al., 2010a, 2010b).
In case of race 2, several resistant PI lines (PI 189225, 203551, 270550, 271775, 271778, 271779, 299379 and 326515) were reported in 1980s (Sowell et al., 1980; Suvanprakorn and Norton, 1980). Boyhan et al. (1994) evaluated 67 PI lines resistant to race 2 and verified previously reported resistant lines and added a new resistant source, PI 512385. Similarly, Patel (2019) evaluated resistance of 1,408 PI germplasms. Suvanprakorn and Norton (1980) reported that the major resistance to race 2 was controlled by a dominant single gene pair, but other genes contributing to resistance were also suggested (Love and Rhodes, 1988).
Morphological traits and disease resistance are important factors for breeding and studies on morphological diversity on genetic resources have been conducted in the past (Desta et al., 2024; Huh et al., 2014; Solmaz and sari, 2009; Szamosi et al., 2009). Study for association of morphological traits and disease resistance were conducted. Iqbal et al. (2024) evaluated brown rust resistance using morphological traits in bread wheat and Zengin et al. (2020) analyzed the relation between morpological traits such as leaf attitude and resistance to tomato yellow leaf curl virus (TYLCV) in tomato. Egea-Gilabert et al. (2008) reported correlation of leaf width and necrosis length of Phytophthora capsici on pepper, leaf angle correlated on brown spot disease severity in rice (Dariush et al., 2020). Especially, relation of anthracnose resistance and morphological traits were studied in common bean (Kiptoo et al., 2020) and sorghum (Mengistu et al., 2020), but not previously reported in watermelon. In this study, we have evaluated anthracnose resistance and morphological traits of 85 watermelon germplasms, followed by investigations on the relationship between resistance to anthracnose and morphological traits.
Materials and Methods
Plant materials and cultivation
Seventy nine sweet watermelon (Citrullus lanatus) and 6 egusi melon (C. mucosospermus) germplasms from the gene bank of the National Agrobiodiversity Center (NAC), Rural Development Administration (RDA), Jeonju, South Korea were used. Six commercial cultivars were used as control. Plants were cultivated at the research field of the NAC, Jeonju, Republic of Korea. Plants were grown following the cultivation manual for watermelon by RDA (RDA, 2022). In brief, seeds were sowed on March 22, 2022, and grown in a nursery bed. Seedlings were grafted on bottle gourd by single cotyledon ordinary splice grafting methods. Ten seedlings were transplanted in a plastic greenhouse on May 03, 2022 with 40 ㎝ × 300 ㎝ spacing. Fruits of the watermelon were harvested 45 days (on average) after pollination at a fully mature stage. Multiplied seeds were used for evaluating resistance to anthracnose.
Morphological traits
The morphological characters were evaluated based on descriptions for watermelon (RDA, 2013) at the physiologically mature stage in the field. Eight quantitative traits (length of leaf, width of leaf, size of pistil scar, weight of fruit, length of fruit, width of fruit, thickness of pericarp and soluble solids contents) were evaluated with help of ruler, digital caliper, digital balance, and a handheld electronic refractometer. Details and measurements of quantitative traits are listed in Table 1. Ten plant samples per each accession were used for the measurement of quantitative morphological characters.
Table 1.
Definition of morphological traits.
Anthracnose inoculum preparation and inoculation
Multiplied seeds from each accessions were sown and used for evaluating resistance to anthracnose. Colletotrichum orbiculare KACC 40809 from Korean Agricultural Culture Collection (KACC) was used to infect the seedlings. The inoculum preparation and inoculation procedure was carried out as described by Correa et al. (2021). In brief, the fungus was grown on potato dextrose agar (PDA) for 12 days. Spores were harvested by adding 2 mL distilled water to each agar plate, rubbing the surface of the agar. Spore suspension was filtered through two layers of cheesecloth into a beaker. Concentration of the inoculum was measured using a hemocytometer and adjusted to 1×105 spores/mL. The spore inoculum was sprayed to 22-days-old watermelon seedlings grown in the growth chamber. After inoculation, seedlings were transferred to a humidity chamber for 48 h in darkness at 90–100% relative humidity (RH), and at a temperature of 25℃. Then, seedlings were moved to a plant growth chamber with 25℃, 60% RH, 12 h light/dark cycle until evaluating the disease index.
Disease index
Disease index (DI) of eight inoculated seedlings were rated at 12 days post inoculation (dpi). The disease index (Fig. 1) was evaluated at a range of 1 to 5 based on percentage of leaf area lesion, adopted from Gichimu et al. (2008). Seedlings with DI≤2 were considered resistant and DI≥4 were considered susceptible.
Statistical analysis
Analysis of variance (ANOVA), followed by the Student–Newman–Keuls tests (α = 0.05), conducted R-program (Version 4.3.3, RStudio, Inc., Boston, MA, USA), was employed to determine the significance of the variation between the mean concentration of each compound between the samples. Correlation analysis and Principal Component Analysis (PCA) also conducted using R-program (Version 4.3.3, RStudio, Inc., Boston, MA, USA).
Results and Discussion
Morphological traits
The variation in quantitative morphological traits in watermelon germplasms are summarized in Table 2. The length and width of leaves were at 28.9±2.3 ㎝ and 30.5±2.9 ㎝, respectively. Weight of fruits ranged at 5955±2248 g, length and width of fruits were 24.0±3.9 ㎝ and 22.3±3.0 ㎝ respectively. Size of pistil scar was at 18.0±9.7 ㎜, thickness of pericarp was 17.8±4.8 ㎜ and soluble solids contents were 8.0±1.6 Brix. Study of morphological traits indicated a wide range of variation, and the highest variation was observed on size of pistil scar (SPS), 53.9%. The lowest variation was observed in leaf length (LL), 8.1%. Assefa et al. (2020) report size of the pistil scar and thickness of the pericarp showed high diversity. Length and width of the leaves were at a range higher than previous studies (Solmaz and Sari, 2009; Szamosi et al., 2009). Size of pistil scar and weight of fruit also had higher values, including the length and width of fruits. Soluble solids contents were similar to the report previous published (Assefa et al., 2020; Solmaz and Sari, 2009; Szamosi et al., 2009). In this study, pericarp was observed to be thicker than previous research study (Assefa et al., 2020; Elbekkay et al., 2021; Huh et al., 2014; Solmaz and Sari, 2009; Szamosi et al., 2009). This difference could be due to the 6 egusi melon (C. mucosospermus) germplasms used in this study, which has thicker pericarp than the other Citrullus species (Achigan-Dako et al., 2015).
Table 2.
Summary statistics of morphological traits of watermelon germplasm.
Parametersz | Minimum | Maximum | Mean | SD | CV (%) |
LL (㎝) | 23.4 | 36.1 | 28.9 | 2.3 | 8.1 |
LW (㎝) | 24.5 | 38.5 | 30.5 | 2.9 | 9.4 |
SPS (㎜) | 5.5 | 53.7 | 18.0 | 9.7 | 53.9 |
WF (g) | 2449.0 | 13570.0 | 5955.0 | 2248.0 | 37.7 |
LF (㎝) | 17.0 | 37.2 | 24.0 | 3.9 | 16.4 |
WIF (㎝) | 14.7 | 31.0 | 22.3 | 3.0 | 13.3 |
TP (㎜) | 9.0 | 38.8 | 17.8 | 4.8 | 26.8 |
SSC (Brix) | 3.2 | 11.2 | 8.0 | 1.6 | 19.8 |
Distribution of disease index
C. orbiculare KACC 40809 were inoculated in 85 accessions which included 4 differential hosts (Charleston Gray, Congo, Fairfax, New hampsher midget). Shim et al. (2013) has reported that pathological races belonging to isolate KACC 40809 estimated race 2, yielded similar results with the this study, which showed that all the differential host’s DI has exceed 2.
Six accessions showed resistance and 77 accessions were found to be susceptible (Fig. 2). Six accessions were distributed in DI≥2 and 77 accessions in DI≤4, only 2 accessions (4.7%) showed moderate resistance. The number of accessions with moderate resistance were low and accessions were divided primarily between resistant and susceptible in this study. These results may stem from resistance to race 1 and 3 (Winstead et al., 1959) and major resistance to race 2 were controlled by a dominant single gene pair (Suvanprakorn and Norton, 1980).
Six accessions which exhibited DI less than 2 are shown in Fig. 3 which includes one accession of C. lanatus and five accessions of C. mucosospermus. Three accessions were found to originate from Nigeria (NGA), 2 from Zaire (ZAR) and 1 from Zambia (ZMB). Watermelon and their wild relatives originated from Africa, especially C. mucosospermus is native to West Africa including Nigeria (Renner et al., 2021). High genetic diversity of crops and wild relatives can be found in the vicinity of center of origin, and in this case, wild relatives of watermelon such as C. amarus (Winstead et al., 1959) and C. colocynthis (Love and Rhodes, 1988) were reported as germplasms of resistance.
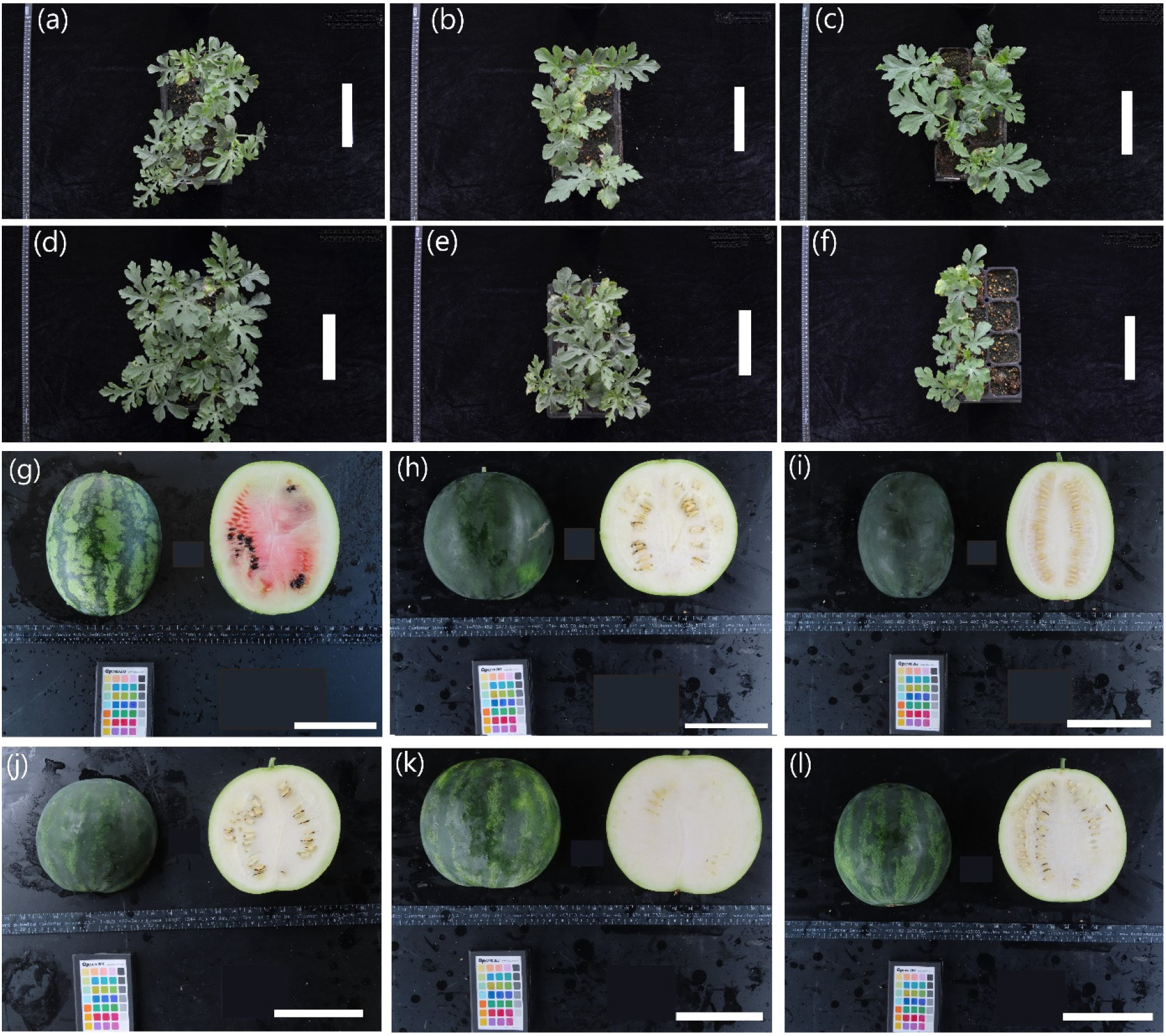
Fig. 3.
Watermelon seedlings (a-f) and their fruit (g-l) which disease index (DI) were below 2.0 at 12 days after inoculation with C. orbiculare KACC 40809, (a, g) K193166 (C. lanantus) from Zambia, DI 1.0 (b, h) K193327 (C. mucosospermus) from Zaire, DI 1.0 (c, i) K193155 (C. mucosospermus) from Nigeria, DI 1.2±0.4 (d, j) K193434 (C. mucosospermus) from Nigeria, DI 1.3±0.5 (e, k) K193436 (C. mucosospermus) from Nigeria, DI 1.6±0.5 (f, l) K193328 (C. mucosospermus) from Zaire, DI 1.8±0.5. Scale bar = 14 ㎝.
Correlation between morphological traits and disease index
Some of the studied characters had a significantly with each other. Table 3 shows correlation between high traits of watermelon germplasms. The length of leaf was significantly positively correlated with width of leaf (r=0.852). Weight of the fruit was also significantly correlated with length of fruit (r=0.718) and width of fruit (r=0.906), length and width of fruit were also significantly correlated (r=0.658) with each other. Soluble solids content (SSC) was correlated with leaf length (r=0.279) and DI (r=0.500). We observed that the DI values significantly correlated to length of leaf (r=0.388), width of leaf (r=0.308) and size of pistil scar (r=0.300). Traits dealing with the leaf size (length and width of leaf) were positively correlated with traits about fruit size (weight, length and width of fruit) which was also statistically significant with each other. Assefa et al. (2020) and Sahin (2024) also reported weight, length and width of fruits correlated with each other, and Achigan-Dako et al. (2015) reported that length and width of leaf were significantly correlated, as well as weight, length and width of fruit were significantly related in his research using four Citrullus species (C. lanatus, C. mucosospermus, C. amarus, and C. colocynthis). Disease severity showed correlation with length and width of leaf, size of pistil scar and soluble solids content in this study. Egea-Gilabert et al. (2008) reported necrosis length of Phytophthora capsici on pepper were correlated with leaf width, resistant plants showed small leaves. Kiptoo et al. (2020) reported anthracnose resistance correlation to leaf length and width in common bean.
Table 3.
Pearson’s correlation coefficient of traits for watermelon genetic resources.
LL | LW | SPS | WF | LF | WIF | TP | SSC | DI | |
LLz | 1 | ||||||||
LWy | 0.852*** | 1 | |||||||
SPSx | 0.123 | 0.008 | 1 | ||||||
WFw | 0.296** | 0.312** | 0.057 | 1 | |||||
LFv | 0.234* | 0.314** | -0.227* | 0.718*** | 1 | ||||
WIFu | 0.131 | 0.134 | 0.080 | 0.906*** | 0.658*** | 1 | |||
TPt | -0.244* | -0.194* | 0.016 | 0.246* | 0.125 | 0.226* | 1 | ||
SSCs | 0.279** | 0.201* | 0.266* | -0.041 | -0.156 | -0.120 | -0.133 | 1 | |
DIr | 0.388*** | 0.308** | 0.300** | 0.241* | -0.033 | 0.175 | -0.111 | 0.500*** | 1 |
Principal component analysis (PCA)
The first three principal components Eigenvalue exceed 1.0 and explained 33.2, 24.8, and 15.2% of the variations, respectively, making a total of 73.2% Fruit weight (25.5%), width (19.6%), length (17.5%) and leaf width (14.8%), length (14.5%) were important variables composing PC1. While PC2 included soluble solid contents (20.3%) and Disease index (13.3%). Size of pistil scar significantly contributed (32.6%) to PC3 (Table 4). Accessions were found to segregate into three groups on the PCA plot (Fig. 4). Cluster 1, 2 and 3 included 46, 9 and 30 accessions, respectively. Variations of traits in three clusters are explained in Fig. 5. In summary, cluster 2 had significantly low value in leaf length (26.2±2.1 ㎝), leaf width (28±2.1 ㎝), disease index (2.2±1.5), size of pistil scar (8.3±2.4 ㎜) and soluble solid content (4.8±1.7 brix). Fruit weight (8380.9±1669.8 g) and fruit width (25.1±2.1 ㎝) were significantly high in cluster 3, whereas cluster 1 and 2 were not significantly different. Fruit lengths were significantly highest in cluster 3 (27.3±3 ㎝), lowest in cluster 1 (21.8±2.3 ㎝). Thickness of pericarp were significantly low in cluster 1 (16.1±3.7 ㎜) than cluster 2 (19.7±3.3 ㎜) and cluster 3 (19.7±5.7 ㎜). Accessions segregated into three clusters, due to all morphological traits showing significant variation. Characteristically, cluster 1 accessions showed low pericarp thickness, cluster 3 accessions had biggest fruit. Accessions in cluster 2 had small leaf, small pistil scar, low SSC and DI. Previous studies reported accessions to exhibit variations in morphological traits (Sahin, 2024; Solmaz and sari, 2009; Szamosi et al., 2009), number of clusters and traits that distinguished the clusters were different. In this study, all of the 6 C. mucosopermus accessions were in cluster 2, which was similar with the previous study where C. mucosospermus accessions were grouped on principal component analysis using C. lanatus, C. colocynthis and C. mucosospermus accessions (Achigan-Dako et al., 2015).
Table 4.
Contribution of traits for principal components, eigenvalues, proportion and cumulative variation.
Descriptorz | PC1 | PC2 | PC3 | PC4 | PC5 |
LL | 14.53171 | 13.25804 | 8.464354 | 2.085066 | 8.685102 |
LW | 14.80356 | 8.788242 | 14.33632 | 5.487421 | 8.005440 |
SPS | 0.375919 | 6.177224 | 32.56144 | 11.85077 | 37.85418 |
WF | 25.52964 | 6.142924 | 2.302304 | 0.846371 | 0.281844 |
LF | 17.48506 | 9.85126 | 3.515809 | 0.188810 | 2.606418 |
WIF | 19.63275 | 9.869231 | 4.870340 | 6.667137 | 0.680365 |
TP | 0.121430 | 11.33471 | 14.79284 | 66.24092 | 5.913225 |
SSC | 0.867541 | 20.35181 | 8.152930 | 6.622847 | 23.39686 |
DI | 6.652401 | 14.22656 | 11.00366 | 0.010663 | 12.57657 |
Eigenvalue | 2.99 | 2.24 | 1.37 | 0.74 | 0.73 |
Proportion | 33.2 | 24.8 | 15.2 | 8.30 | 8.10 |
Cumulative | 33.2 | 58.0 | 73.2 | 81.5 | 89.6 |
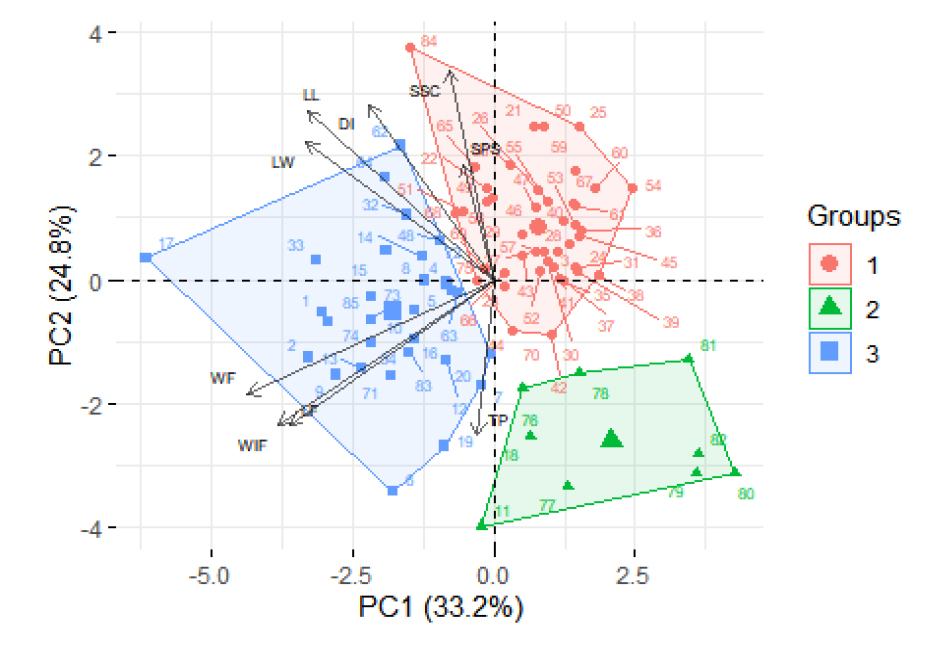
Fig. 4.
Principal Component Analysis (PCA)-Biplot of watermelon germplasms with morphological characters measured quantitatively. SPS = Size of pistil scar (mm); WS = Width of stripes (mm); WF = Weight of fruit (kg); LF = Length of fruit (cm); WIF = Width of fruit (cm); TP = Thickness of outer layer of pericarp (mm); SSC = Soluble solids content (Brix). DI = Disease index.
In conclusion, this study evaluated the resistanceto anthracnose and quantitative morphological traits of 85 watermelon accessions and their correlation with each other. Six accessions from Africa showed resistance, which included one accession of C. lanatus, and five from C. mucosospermus. Accessions which showed resistance to anthracnose characteristically had small leaf, pistil scar and low soluble solids content. Resistance accessions and their characteristics observed in this study will provide the preliminary data on selecting the resources in further research for anthracnose resistance and morphological traits in watermelon.