Introduction
Materials and Methods
Reagents
HMF preparation
Cell culture
Measurement of cell viability
Measurement of NO
SDS-PAGE and Western blot analysis
Reverse transcriptase-polymerase chain reaction (RT-PCR)
Statistical analysis
Results and Discussion
Effect of HMF on macrophage activation in RAW264.7 cells
Effect of TLR 2 and 4 on HMF-mediated increase of immunostimulatory factors in RAW264.7 cells
Effect of MAPK signaling pathway on HMF-mediated increase of immunostimulatory factors in RAW264.7 cells
Introduction
Immunity is an important countermeasure to protect the human body from various diseases caused by invasion of foreign pathogens. In particular, with the current global COVID-19 pandemic, which threatens human life, the functionality and effectiveness of the immune response is considered important in combating viral infections (Alagawany et al., 2021). Among various immune cells, macrophages, which play an important role in the innate immune system, are known to protect the human body from foreign pathogens through phagocytosis (Duque and Descoteaux, 2014; Tosi, 2005). In addition, macrophages secrete nitric oxide (NO), inducible nitric oxide synthase (iNOS), interleukin-1β (IL-1β), interleukin-6 (IL-6) and tumor necrosis factor-α (TNF-α), which activates T-cells and B-cells responsible for the acquired immune response (Duque and Descoteaux, 2014; Tosi, 2005). Therefore, it is reported that macrophages are involved not only in the innate immune response but also in the acquired immune response. Since macrophages are involved in all immune systems, activation of macrophages is considered as an important target that can fundamentally block the development of diseases caused by foreign pathogens (Mills and Ley, 2014; Mills et al., 2015).
Hibiscus manihot is a herbaceous plant known as an edible herb belonging to the family Malvaceae. Through recent studies, Hibiscus manihot has been reported to have various pharmacological properties such as anticonvulsant, antioxidant, anti-inflammatory, analgesic, antidepressant, antiviral, antitumor, antiplatelet, anti-Crohn's disease, post-stroke depression, angiogenesis promotion, cardioprotection, neuroprotection, immune regulation, hepatoprotecion, cerebral infarction, and anti-bone loss (Luan et al., 2020). Although the immunostimulatory activity of Hibiscus manihot has been reported (Pan et al., 2018; Yu et al., 2021), studies on the mechanism of action are insufficient. Therefore, in this study, the immunostimulatory activity and mechanism of action of Hibiscus manihot flower was evaluated.
Materials and Methods
Reagents
Dulbecco's Modified Eagle medium (DMEM)/F-12 1:1 Modified medium was purchased from Lonza (Morristown, NJ, USA). 3-(4,5-dimethylthizaol-2-yl)-2,5-diphenyl tetrazolium bromide (MTT), C29 (TLR2 inhibitor), TAK-242 (TLR4 inhibitor), PD98059 (ERK1/2 inhibitor), SB203580 (p38 inhibitor) and SP600125 (JNK inhibitor) were purchased from Sigma-Aldrich (St. Louis, MO, USA). Primary and secondary antibodies were purchased from Cell Signaling Technology (Danvers, MA, USA).
HMF preparation
Hibiscus manihot flowers (voucher number: ANU-2021-002) were provided from Agricultural Corporation E-Farm Corp., Andong, Korea after botanical identification through Forest Medicinal Resources Research Center, National Institute of Forest Science, Yongju, Korea. To prepare the HMF, 20 g of Hibiscus manihot flowers were immersed in 400 mL distilled water and then extracted at 40℃ for 12 h. After 12 h, the extracts were centrifuged at 12,000 rpm for 10 min at 4℃ to obtain a clear supernatant. Then, the recovered supernatant was lyophilized. Freeze-dried Hibiscus manihot flowers (HMF) were stored at -80℃ until use. HMF was dissolved in sterile distilled water, and aliquoted in small portions, and stored at -80℃ until use.
Cell culture
RAW264.7 cells (mouse macrophages) were purchased from American Type Culture Collection (Manassas, VA, USA). RAW264.7 cells were cultured in Dulbecco's Modified Eagle medium (DMEM)/F-12 1:1 Modified medium (Lonza, Walkersville, MD, USA) containing 10% fetal bovine serum, 100 U/mL penicillin and 100 ㎍/mL streptomycin at 37℃ under a humidified atmosphere of 5% CO2.
Measurement of cell viability
Since the MTT assay is an experimental method applied to evaluate cytotoxicity and cell viability, we performed the MTT assay to evaluate the cytotoxicity of HMF against RAW264.7 cells. The cells cultured in a 96-well plate for 24 h were treated with HMF by concentrations, and re-cultured at 37℃ under a humidified atmosphere of 5% CO2 for the additional 24 h. Then, after treatment with 50 μL of MTT solution (1 ㎎/mL) for 4 h, the cell culture medium was removed, and the resulting crystals were eluted from cells by treating each well with 100 μL of DMSO. Then the absorbance of the eluted resulting crystals was measured at 570 ㎚ using UV/Visible spectrophotometer (Human Cop., Xma-3000PC, Seoul, Korea).
Measurement of NO
Griess assay was applied to evaluate the effect of HMF on NO production in RAW264.7 cells. RAW264.7 cells plated in a 12-well plate for 24 h were treated with HMF by concentrations and cultured at 37℃ under a humidified atmosphere of 5% CO2 for the additional 24 h. Then, the cell culture media and Griess solution (Sigma Aldrich) were mixed at a ratio of 1:1 and allowed to stand at room temperature for 15 min, and the absorbance was measured at 540 ㎚ using UV/Visible spectrophotometer (Human Cop., Xma-3000PC, Seoul, Korea).
SDS-PAGE and Western blot analysis
The samples for SDS-PAGE were prepared by extracting proteins from 3T3-L1 cells with RIPA buffer (Boston Bio Products, Ashland, MA, USA) containing protease inhibitor (Sigma-Aldrich) and phosphatase inhibitor (Sigma-Aldrich) and quantifying the protein amounts by BCA protein assay (Thermo Fisher Scientific, Waltham, MA USA). After sample preparation, SDS-PAGE was performed for protein separation, and then proteins separated on the gel were transferred to the PVDF membrane. After the transfer, the PVDF membrane was blocked with 5% nonfat milk in Tris-buffered saline containing 0.05% Tween 20 (TBS-T) at room temperature for 1 h, and then the PVDF membranes were treated with the primary antibodies in 5% BSA in TBS-T at 4℃ for overnight. After the primary antibody treatment was completed, the PVDF membranes were treated with the secondary antibodies in 5% nonfat milk in TBS-T at room temperature for 1 h. Chemiluminescence was detected with ECL Western blotting substrate (Amersham Biosciences, Piscataway, NJ, USA) and visualized using LI-COR C-DiGit Blot Scanner (Li-COR Biosciences, Lincoln, NE, USA).
Reverse transcriptase-polymerase chain reaction (RT-PCR)
The isolation of mRNA from RAW264.7 cells and the synthesis of cDNA using the isolated mRNA were carried out using a RNeasy Mini Kit (Valencia, CA, USA) and a Verso cDNA Kit (Thermo Scientific, Pittsburgh, PA, USA), respectively. The amplification of the target gene was performed using a PCR Master Mix Kit (Promega, Madison, WI, USA) and the primers. The sequences of the primers used in this study were as follows : iNOS: forward 5’-ttgtgcatcgacctaggctggaa-3’ and reverse 5’-gacctttcgcattagcatggaagc-3’, IL-1β: forward 5’-ggcaggcagtatcactcatt-3’ and reverse 5’-cccaaggccacaggtattt-3’, TNF-α: forward 5ʹ-tggaactggcagaagaggca-3ʹ and reverse 5ʹ-tgctcctccacttggtggtt-3ʹ, GAPDH: forward 5’-ggactgtggtcatgagcccttcca-3’ and reverse 5’-actcacggcaaattcaacggcac-3’. The PCR results were visualized using agarose gel electrophoresis.
Statistical analysis
All the data are shown as mean ± SD (standard deviation). Statistical significance was determined by Student’s t-test. Differences with *P < 0.05 and #P < 0.05 were considered statistically significant.
Results and Discussion
Effect of HMF on macrophage activation in RAW264.7 cells
Macrophages are known to be involved in inflammation regulation and immune enhancement (Kasimu et al., 2017). From the standpoint of immune enhancement, activation of macrophages produces various immunostimulatory factors such as NO, iNOS, IL-1β, and TNF-α to eliminate foreign pathogens (Ma et al., 2016; Siveen and Kuttan, 2009). It is known that NO secreted from macrophages activated by invading foreign pathogens directly destroy foreign pathogens (Nathan and Shiloh, 2000; Foley and O’Farrell, 2003). It has been reported that IL-1β contributes to increased antigen- presenting activity of macrophages, increased viability of T-cells, and activation of various innate immune cells (Seillet et al., 2014). In addition, TNF-α has been reported to increase phagocytosis so that macrophages can eliminate foreign pathogens (Barbara et al., 1996). Therefore, various immunostimulatory factors secreated by activated macrophages play an important role in host defense against the foreign pathogens. Thus, natural agents that increase the secretion of immunostimulatory factors such as NO, iNOS, IL-1β, and TNF-α are considered to have potential immune enhancing activity.
To confirm whether HMF activates macrophages, we measured level of immunostimulatory factors such as NO, iNOS, IL-1β, and TNF-α in HMF-treated RAW264.7 cells. As shown in Fig. 1A, HMF significantly increased NO level in RAW264.7 cells. In addition, the expression of immunostimulatory factors such as iNOS, IL-1β, and TNF-α was up-regulated in HMF- treated RAW264.7 cells (Fig. 1B). These results suggest that increase of immunostimulatory factors secreted from macrophages by HMF may contribute to enhancing the immune response of the human body. 100 Fig. 1. Effect of HMF on macrophage activation in RAW264.7 cells. RAW264.7 cells were treated with HMF for 24 h. NO level (A) and mRNA level of immunostimulatory factors (B) were measured by Griess assay and RT-PCR, respectively. *P < 0.05 compared to the cells without the treatment.
Effect of TLR 2 and 4 on HMF-mediated increase of immunostimulatory factors in RAW264.7 cells
Macrophage toll-like receptors (TLRs), one of the pattern- recognition receptors that recognize pathogen-associated molecular patterns of foreign pathogens, are known to play a role in initiation an innate immune response by secreting immunostimulatory factors (Janeway and Medzhitov, 2002) In addition, it has been reported that TLRs are involved in the preparation and coordination of the acquired immune response, which is an antigen-specific immune response (Janeway and Medzhitov, 2002). Thus, these roles of TLRs are believed to be essential in eliminating foreign pathogens (Kawasaki and Kawai, 2014). Recently, among TLRs, TLR2 and TLR4 has been reported as an important target for the development of immune adjuvants (Toussi and Massari, 2014). Lipopolysaccharide (LPS) stimulating TLR4 has strong immunostimulatory activity, so many vaccine clinical studies have been conducted for its use as an immune adjuvant, but its use has been limited due to its intrinsic toxicity (Toussi and Massari, 2014).
To evaluate whether TLR2 and TLR4 are involved in the production of immunostimulatory factors by HMF, RAW264.7 cells were pretreated with C29 (TLR2 inhibitor) and TAK-242 (TLR4 inhibitor), co-treated with HMF, and then measured the level of immunostimulatory factors such as NO, iNOS, IL-1β, and TNF-α. As shown in Fig. 2A, the production of NO induced by HMF was suppressed in the presence of C29 and TAK-242. In addition, the treatment of C29 and TAK-242 inhibited the expression of iNOS and IL-1β, but not TNF-α (Fig. 2B). These results suggest that since HMF stimulates TLR2 and TLR4 to induce the secretion of NO, iNOS, and IL-1β, HMF can be used as a natural agent for the development of immune adjuvants.
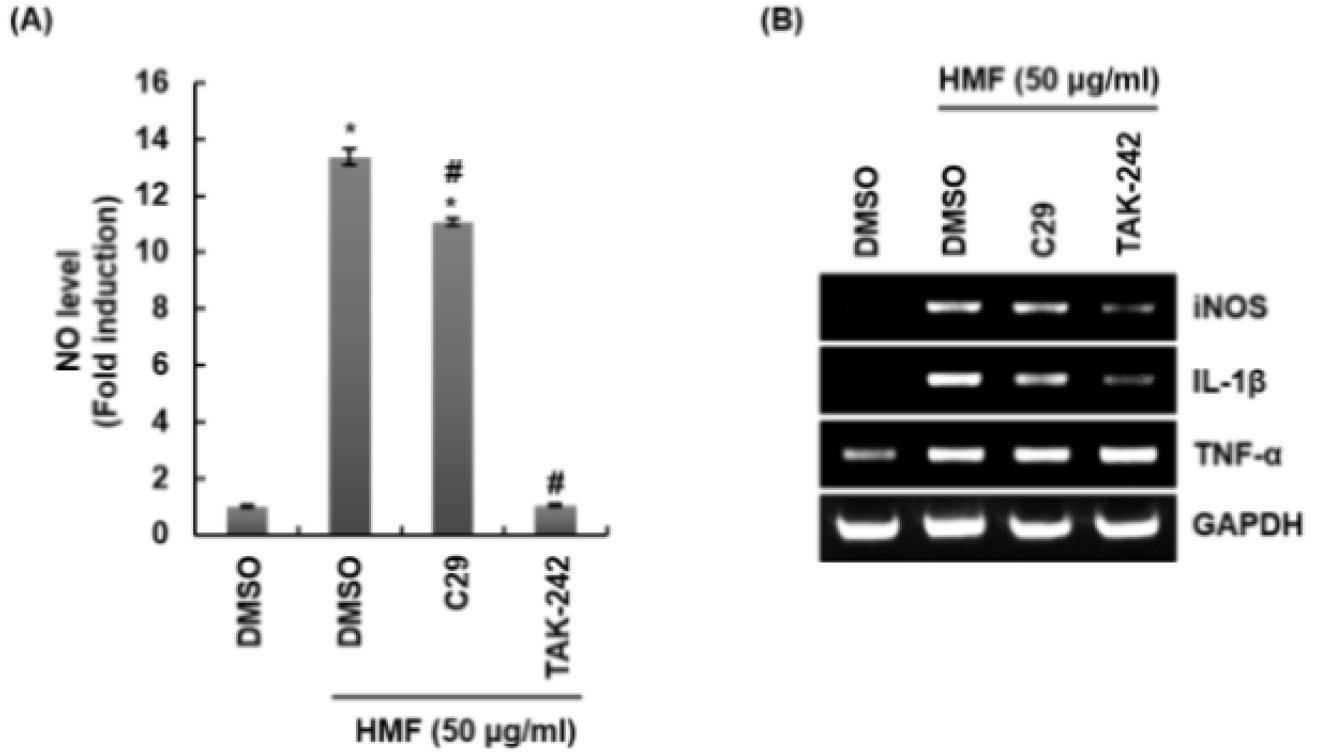
Fig. 2.
Effect of TLR2 and TLR4 on the production of immunostimulatory factors in HMF-treated RAW264.7 cells. RAW264.7 cells were pre-treated with C29 (TLR2 inhibitor, 100 μM) or TAK-242 (TLR4 inhibitor, 5 μM) for 2 h and co-treated with HMF (50 ㎍/mL) for 24 h. NO level (A) and mRNA level of immunostimulatory factors (B) were measured by Griess assay and RT-PCR, respectively. *P < 0.05 compared to the cells without the treatment. #P < 0.05 compared to the cells with HMF alone.
Effect of MAPK signaling pathway on HMF-mediated increase of immunostimulatory factors in RAW264.7 cells
Mitogen-activated protein kinases (MAPKs) activation has been reported to participate in the initiation of the immune response of macrophage and the preparation and coordination of the acquired immune response by TLRs signaling (Reiling et al., 2001; Zhang et al., 2013). MAPKs consist of three types (ERK1/2, p38 and JNK), which are known to be activated simultaneously or independently (Wada and Penninger, 2004). These MAPKs have been demonstrated to be important in the secretion of immunostimulatory factors such as NO, iNOS, IL-1β, and TNF-α in macrophages caused by TLRs (Nick et al., 1996).
To investigate whether the MAPK signaling pathway affects the production of immunostimulatory factors induced by HMF, the levels of NO, iNOS, IL-1β, and TNF-α produced by macrophages were measured after treatment with MAPK signaling inhibitors and HMF. As shown in Fig. 3A, NO production by HMF was inhibited in the presence of SB203580 (p38 inhibitor) and SP600125 (JNK inhibitor), but not PD98059 (ERK1/2 inhibitor). In addition, iNOS expression by HMF was attenuated in presence of PD98059 and SP600125 and IL-1β expression by HMF was blocked with the treatment of SB203580. TNF-α expression by HMF was slightly inhibited in presence of PD98059 (Fig. 3B). To investigate whether HMF activates MAPK signaling pathway, RAW264.7 cells were treated with HMF and then the phosphorylation level of ERK1/2, p38 and JNK was checked by Western blot analysis. As shown in Fig. 3C, it was found that the phosphorylation of ERK1/2, p38 and JNK was increased by HMF treatment, which indicates that HMF may activate MAPK signaling pathway. These results suggest that MAPKs signaling is involved in HMF-mediated production of immunostimulatory factors although ERK1/2, p38 and JNK act independently in HMF- mediated production of immunostimulatory factors.
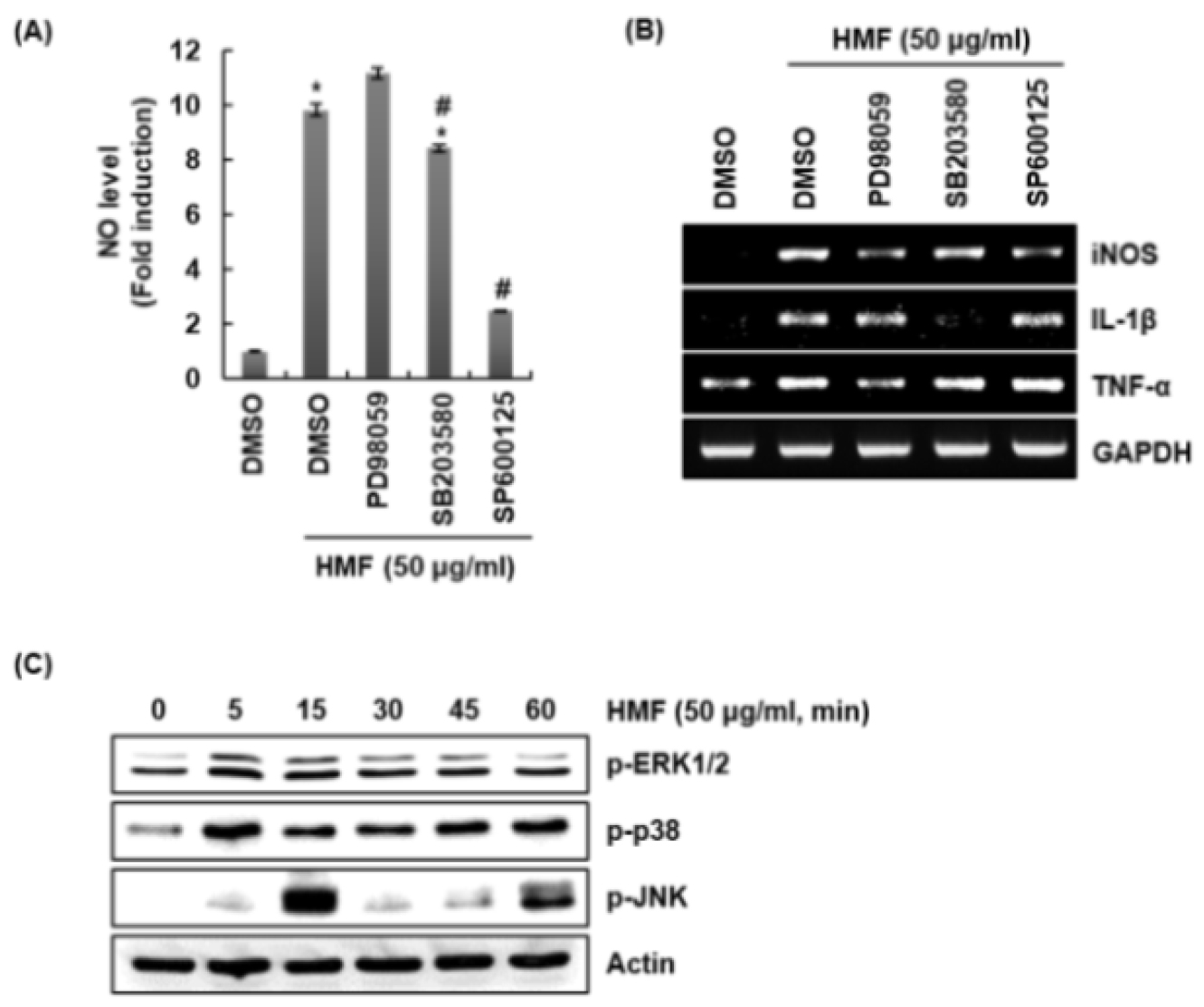
Fig. 3.
Effect of MAPK signaling pathway on the production of immune enhancing factors in HMF-treated RAW264.7 cells. RAW264.7 cells were pretreated with PD98059 (ERK1/2 inhibitor, 40 μM), SB203580 (p38 inhibitor, 40 μM) or SP600125 (JNK inhibitor, 40 μM) for 2 h and then co-treated with HMF (50 ㎍/mL) for 24 h. NO level (A) and mRNA level of immunostimulatory factors (B) were measured by Griess assay and RT-PCR, respectively. *P < 0.05 compared to the cells without the treatment. #P < 0.05 compared to the cells with HMF alone. (C) RAW264.7 cells were treated with HMF (50 ㎍/mL) for the indicated times. Protein levels were measured by Western blot analysis.
Considering the overall results related to immune enhancement, HMF is thought to promote the production of immunostimulatory factors through activating TLR2/4/MAPK signaling in macrophages. It is believed that HMF can be developed as an agent related to immune enhancement in the future. However, animal-based preclinical studies related to HMF’s immune enhancement are required since this study was cell-based.