Introduction
Materials and Methods
Sample preparation
Cell culture
NO and lactate dehydrogenase (LDH)-release assay
Western blot analysis
Statistical analysis
Results
Effects on cell viability of LFE and JLS in RAW 264.7 cells
Effects on pro-inflammatory mediator (NO, iNOS, and COX-2) of LFE and JLS in LPS-stimulated RAW 264.7 cells
Effects of MAPK and NF-κB expressions of LFE and JLS in LPS-stimulated RAW 264.7 cells
Discussion
Introduction
Inflammation is induced by inflammatory or pro-inflammatory cytokines as a complex response to infection and injury (Medzhitov, 2008; Zhang and Wang, 2014). While this process functions as a critical protective reaction against physiological damage, prolonged inflammation can cause a variety of chronic inflammatory disease, such as arthritis (Pawelec et al., 2014; Tsoupras et al., 2018). Macrophages are activated by lipopolysaccharides (LPS) from bacterial outer membranes and produce pro-inflammatory cytokines including tumor necrosis factor (TNF-α), interleukin (IL)-1β, and mediators such as nitric oxide (NO), prostaglandin, and histamine (Abdulkhaleq et al., 2018; Ljung et al., 2006). Cyclooxygenase-2 (COX-2) and nitric oxide synthase (iNOS) are enzymes involved in the synthesis of NO and Prostaglandin E2 (PGE2) (Surh et al., 2001). LPS induced NO production and cytokine secretion in murine macrophage RAW 264.7. Furthermore, LPS-stimulated RAW 264.7 cells increased the expression of nuclear factor-κB (NF-κB) (Guijarro-Muñoz et al., 2014) and mitogen-activated protein kinase (MAPK) family proteins (Ciesielska et al., 2021; Wijayanti et al., 2004).
Jeju Lava Seawater (JLS) is produced in the eastern part of Jeju, South Korea as drinking water. JLS has been purified by natural volcanic rocks and contains various minerals including magnesium (Mg), calcium (Ca), selenium (Se), potassium (K), zinc (Zn), etc. (Jung et al., 2017; Woo et al., 2008). Supplemental Ca and Mg have been shown to exert beneficial influences on human health, such as preventing or managing inflammation-related disease (Mazur et al., 2007; Nielsen, 2010; Pittas et al., 2007). Litsea japonica fruit is plant used to create a traditional herbal medicine applied to treat bone pain, inflammation, and asthma (Kong, 2015). The derived material is known to be rich in lactones, terpenoids, and fatty acids (Min et al., 2003; Ngo et al., 2018; Tanaka et al., 1990). Prior studies demonstrated that Litsea japonica fruit extract (LFE) exerts anti-inflammation and anti-osteoarthritis activities via reduction of inflammatory cytokines or inhibition of MAPK signaling (Jeong et al., 2015). In this study, we investigated the synergistic anti-inflammatory effect of cotreatment with LFE and JLS to inhibit NF-κB and MAPK expression in LPS-stimulated RAW 264.7 cells.
Materials and Methods
Sample preparation
JLS was provided by Yong-am-hae-su Center (Jeju, Korea). JLS was prepared with a Ca:Mg ratio of 2:1 and hardness level of 1,000 ㎎/L. Then, 10 g of the dried Litsea japonica fruit were soaked in 50% ethanol (1:20 w/v) and extracted for 24 h at room temperature at 200 rpm. The ethanolic extracts were filtered through filter paper, evaporated using a rotary evaporator at 40℃, and freeze-dried to collect the extract powder (4.72 g).
Cell culture
The murine macrophage cell line, RAW 264.7 cells, was purchased from American Type Culture Collection and maintained in Dulbecco’s Modified Eagle’s medium (DMEM, Gibco) supplemented with 10% Fetal Bovine Serum (FBS, Gibco), 100 U/mL penicillin, and 100 ㎍/mL streptomycin (Gibco). The cells were cultured in a humidified incubator at 37℃ and 5% CO2.
NO and lactate dehydrogenase (LDH)-release assay
After RAW 264.7 cells were incubated at a density of 1.5 × 105 cells/mL for 18 h, they were subjected to varying concentrations (0, 5, 10, and 20 ㎍/mL) of LFE in the presence or absence of JLS for 24 h. Nitrite levels in the culture medium was assessed to as an indicator of NO production. Then, 100 μL of the culture medium was mixed with 100 μL of the Griess reagent (1% sulfanilamide and 0.1% N-(1-Naphthyl)ethylenediamine dihydrochloride in 2.5% phosphoric acid), and the mixture was incubated at room temperature for 10 min. 2-amino-4-methylpyridine (2A4M, Sigma-Aldrich) was used as a positive control. The absorbance was measured using a microplate reader at 540 ㎚. A LDH release assay kit (Promega) was used according to the manufacturer’s instructions.
Western blot analysis
RAW 264.7 cells were exposed to 10 ㎍/mL of LFE and/or JLS upto 24 h. The cells were then lysed using Mammalian Protein Extraction Reagent lysis buffer (Gibco) supplemented with protease and phosphatase inhibitors. Subsequently, protein concentrations were determined using a Qubit4 fluorometer. Equal amounts of proteins were separated on sodium dodecyl sulfate (SDS)-polyacrylamide gels (Invitrogen) ranging from 8% to 12% and transferred onto a polyvinylidene difluoride (PVDF) membrane (Bio-Rad Laboratories). The membranes were blocked with a solution of 3% bovine serum albumin (BSA, Gibco) in Tris-buffered saline with 0.1% Tween20 detergent (TBST), and incubated with primary antibodies (iNOS, COX-2, p-ERK, ERK, p-JNK, JNK, p-p38, p38, p-IĸB-ɑ, IĸB-ɑ, p-p65, p65, Cell Signaling Technology, and β-actin, Sigma-Aldrich) diluted at a ratio of 1:1,000 in TBS overnight at 4℃. After washing three times with TBST, the membranes were incubated with the corresponding secondary antibodies (Cell Signaling Technology) at a dilution of 1:4,000 at room temperature for 2 h. The visualization of protein bands was achieved using an enhanced chemiluminescence (ECL) kit with a Vilber Western blot imaging system.
Statistical analysis
The data is displayed in the format of mean ± standard deviation (SD). Variations among multiple groups were assessed through one-way analysis of variance. A significance level of P < 0.01 was deemed statistically significant.
Results
Effects on cell viability of LFE and JLS in RAW 264.7 cells
We first examined the viability of LFE and JLS in RAW 264.7 cells. Cells were treated with varying concentrations of LFE or JLS for 24 h. The results demonstrated that LFE and JLS did not significantly impact on cell viability at concentrations of 2.5, 5, 10, 20, and 40 ㎍/mL for LFE and 2.5, 5, and 10% for JLS for 24 h (Fig. 1).
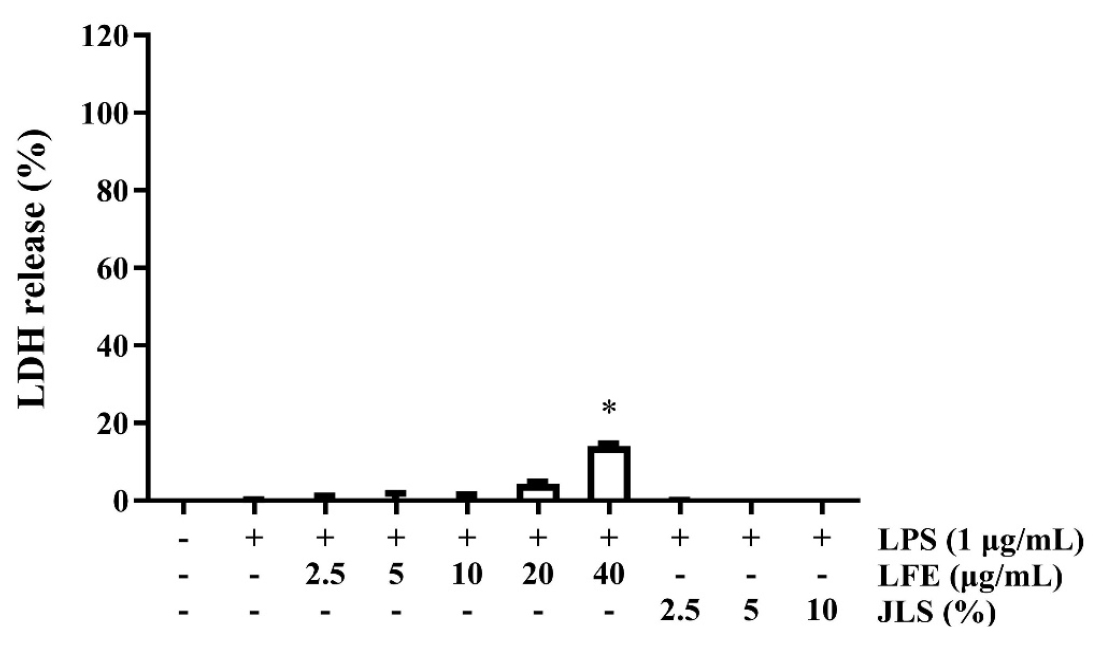
Fig. 1.
Effect of LFE on cell viability. RAW 264.7 cells were incubated for 24 h with different concentrations of LFE (2.5, 5.0, 10.0, 20.0, 40.0 ㎍/mL) or JLS (2.5, 5, 10%) with or without 1 ㎍/mL of LPS. Cell viability was measured using LDH-release assay. Data was presented as mean ± SD (n=3). * p < 0.01 vs. non-treated control.
Effects on pro-inflammatory mediator (NO, iNOS, and COX-2) of LFE and JLS in LPS-stimulated RAW 264.7 cells
To determine the anti-inflammatory activity of cotreatment with LFE and JLS in RAW 264.7 cells, a Griess assay was performed. The combination treatment of 2.5, 5, and 10 ㎍/mL for LFE and 2.5, 5, and 10% for JLS did not affect cell viability (Fig. 2A), yet cotreatment of 20 ㎍/mL for LFE and 2.5, 5, and 10% for JLS increased LDH release by 3.5±0.1%, 15.0±0.6%, and 21.8±1.3% (data not shown). LPS-stimulated NO production was inhibited in a dose-dependent manner. In particular, individual or cotreatment of 10 ㎍/mL of LFE and various concentrations of JLS inhibited NO production by 73.8±1.9%, 67.2±2.0%, 64.9±4.4%, and 45.8±0.8% (0, 2.5, 5, and 10%, respectively; Fig. 2B).
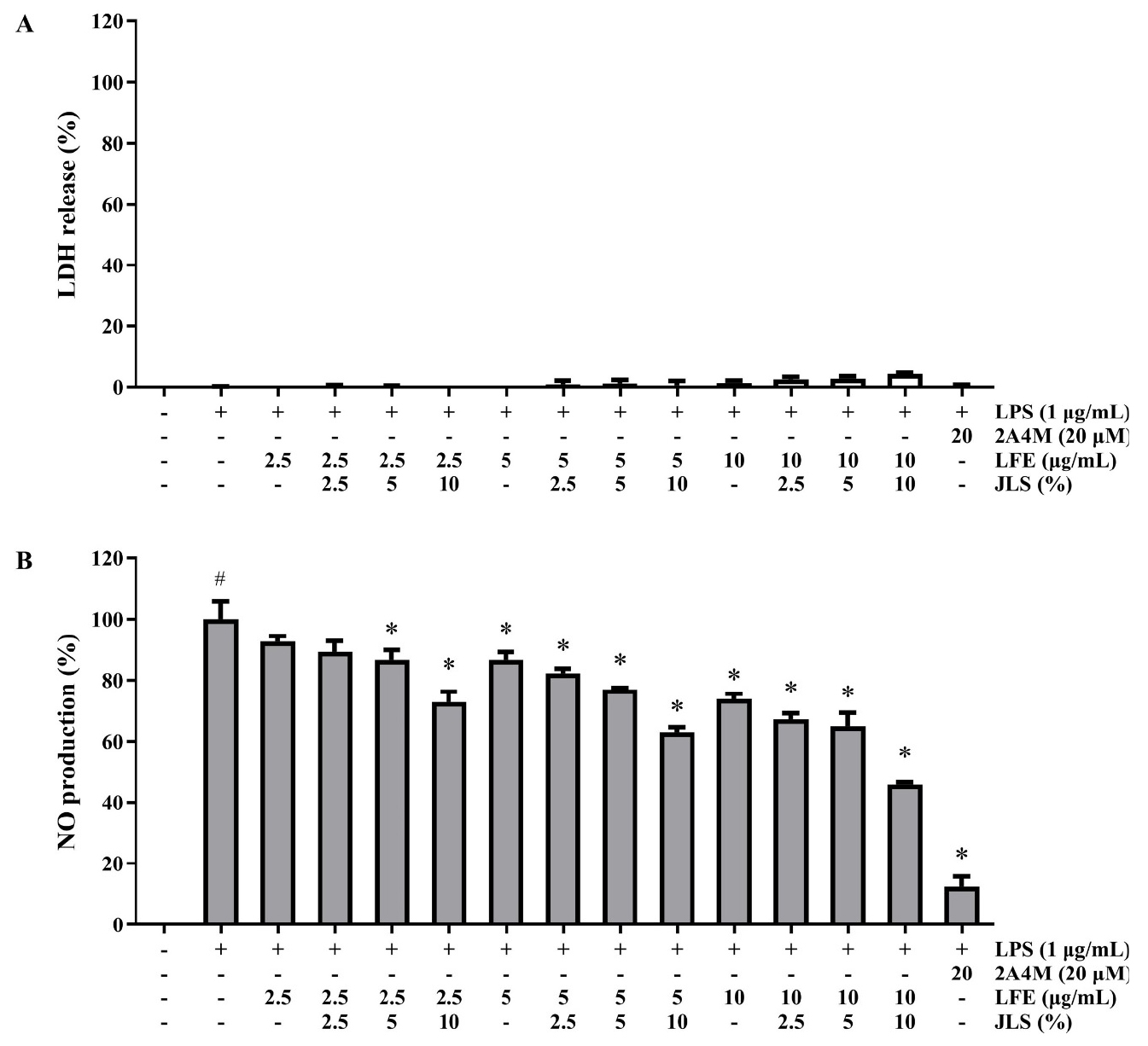
Fig. 2.
Effects of LFE and JLS cotreatment on cell viability (A) and NO production (B). The cells were incubated with different concentrations of LFE (2.5, 5, 10 ㎍/mL) and JLS (2.5, 5, 10%) with or without 1 ㎍/mL of LPS. The cell viability and NO production were measured using LDH-release assay and NO production. Data was presented as mean ± SD (n=3). # p < 0.01 vs. non-treated control; * p < 0.01 vs. LPS-only treated group.
We conducted western blot analysis to investigate whether cotreatment with LFE and JLS affected iNOS and COX-2 expression. The results showed that LPS induced high COX-2 and iNOS expression. The combination treatment downregulated COX-2 and iNOS expression in a concentration-dependent manner. The results demonstrated that the combination treatment decreased NO production by reducing LPS-stimulated COX-2 and iNOS expression in RAW 264.7 cells (Fig. 3).
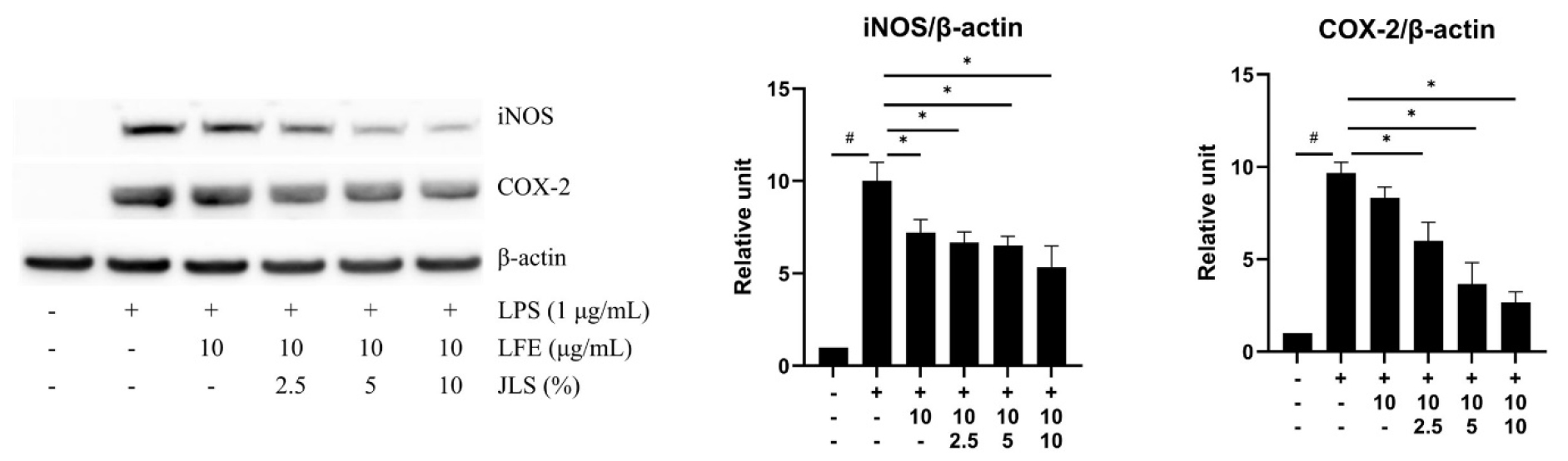
Fig. 3.
Effects of LFE and JLS cotreatment on iNOS and COX-2 expression . Cells were treated with indicated concentrations of LFE and JLS for 24 h. Protein expression was quantified by ImageJ after western blot. Western blot analysis and quantification of the iNOS, COX-2, β-actin. Data was presented as mean ± SD (n=3). # p < 0.01 vs. non-treated control; * p < 0.01 vs. LPS-only treated group.
Effects of MAPK and NF-κB expressions of LFE and JLS in LPS-stimulated RAW 264.7 cells
To characterize the signaling pathway underlying cotreatment with LFE and JLS, we examined the phosphorylation levels of MAPKs. LPS induced phosphorylation of ERK, JNK, and p38. Combined treatment with LFE and JLS reduced the expression of p-ERK, p-JNK, and p-p38 (Fig. 4A). LPS increased the phosphorylation of NF-κB-p65 and IκB-α and degradation of IκB-α. Cotreatment with LFE and JLS suppressed the phosphorylation of p65 and IκB-α. Degradation of IκB-α was mildly inhibited by cotreatment with LFE and JLS (Fig. 4B).
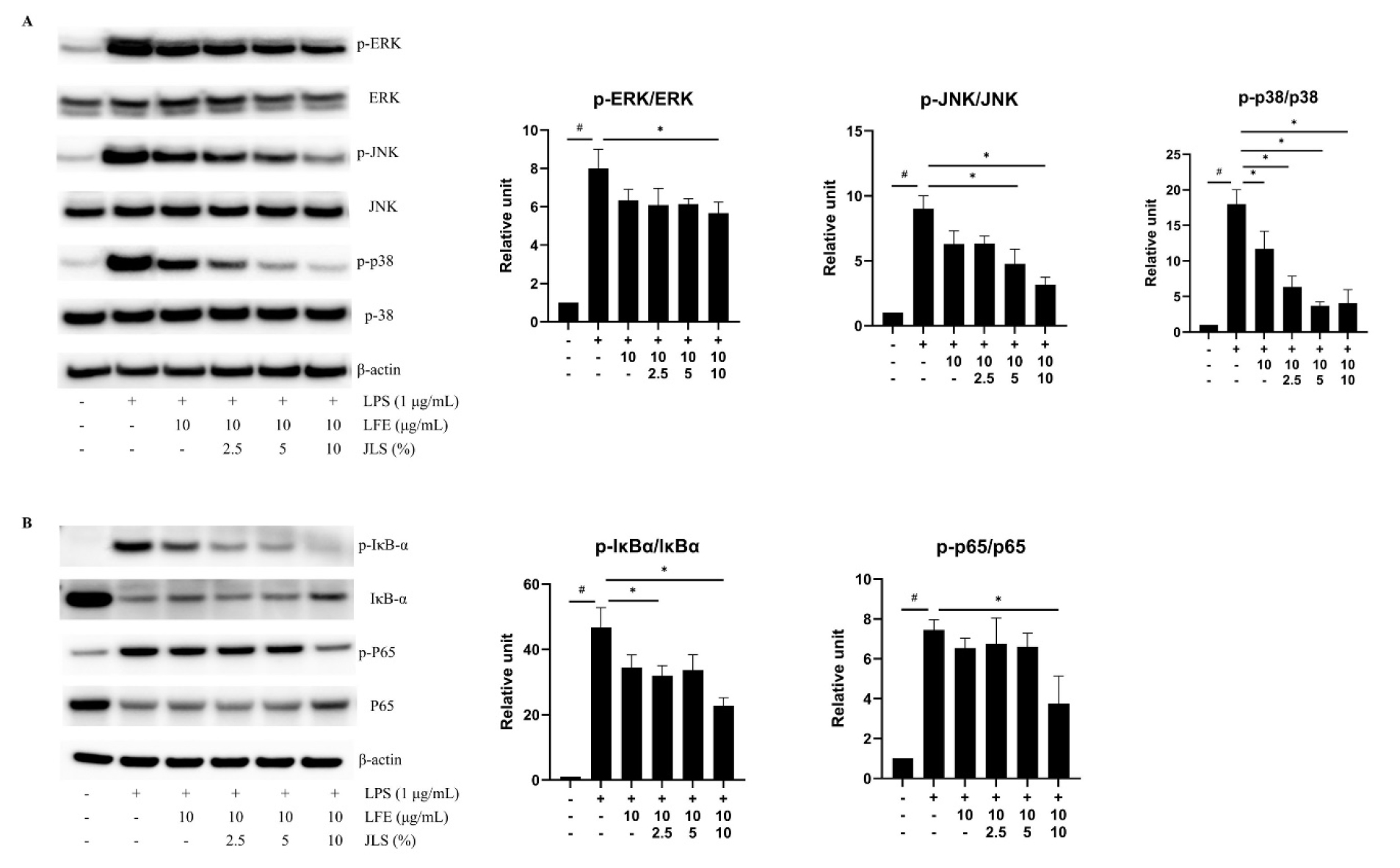
Fig. 4.
Effects of LFE and JLS cotreatment on MAPK and NF-κB expression. Cells were treated with the indicated concentrations of LFE and JLS for 10~30 min depending on the protein. Protein levels in the phosphorylated and total forms were quantified using ImageJ software after western blotting. Western blot analysis and quantification of the: (A) p-ERK, ERK, p-JNK, JNK, p-p38, p-38, and β-actin; (B) p-IĸB-ɑ, IĸB-ɑ, p-p65, p65, and β-actin. Data was presented as mean ± SD (n=3). # p < 0.01 vs. non-treated control; * p < 0.01 vs. LPS-only treated group.
Discussion
This study focused on the benefits of JLS, which enhanced the anti-inflammatory activity of LFE following our previous work, which demonstrated the anti-inflammatory effects of LFE crude extract (Namkoong et al., 2015; Koo et al., 2014; Yoon et al., 2015), solvent fraction (Ham et al., 2017), and supercritical extract (Song et al., 2016). Hard water, known for its high minerals, demonstrated beneficial effects in managing diseases such as diabetes, obesity, cancer, and skin problems by supplying trace elements (Choi et al., 2015; Mohd Nani et al., 2016; Sengupta, 2013). Chun et al. (2017) reported that deep-sea water regressed the inflammatory responses including production of inflammatory meditors. Moreover, several reports point out that JLS may have beneficial synergistic effects on skin moisturizing, antioxidation, anti-inflammation, and antiobesity recently (Heo et al., 2024; Hyun et al., 2021; Kim et al., 2024; Lee et al., 2016).
The overproduction and accumulation of NO are indicators of inflammation and inflammatory diseases. NO is generated from L-arginine by iNOS, endothelial nitric oxide synthase (eNOS), or neuronal NOS (nNOS) (Cinelli et al., 2020). Continued high levels of NO and PGE2 are related to acute and chronic diseases via iNOS and COX-2 expressions (Bengmark, 2006). The signaling pathway from MAPK to the NF-κB, mediating the inflammatory response, has been reported in different inflammatory conditions. MAPKs have been implicated in the signaling cascade triggered by LPS-stimulated inflammation in mouse macrophages (Li et al., 2022). ERK1/2, JNK, and p38 are major molecules leading expression of proinflammatory cytokines and inflammatory mediators such as TNF-α, iNOS, COX-2, NO, etc (Kaminska, 2005; Tang et al., 2016; Yang et al., 2000). We found that the concurrent administration of LFE and JLS in presence of LPS significantly inhibited the production of NO by inhibiting iNOS and COX-2 expressions in RAW 264.7 comparing to the LFE-only group. The combined treatment with LFE and JLS in the presence of LPS strongly inhibited ERK, JNK, and p38 phosphorylation. Each MAPK has a specific role in regulating NO production. JLS and LFE carried out experiments to inhibit three MAPKs involved in the inflammatory process. NF-κB also serves also as a crucial transcription factor involved in inflammatory processes in macrophage. Comprising a heterodimer of NF-κB (p65 and p50), NF-κB forms a complex with IκB-α (Cao, 2016). LPS stimulation causes rapid phosphorylation and degradation of IκB-α following the liberation of NF-κB, (Espinosa et al., 2003). Then, NF-κB relocates to the nucleus and initiates the inflammatory process by acting as a transcription factor (Zinatizadeh et al., 2021).
In conclusion, we demonstrated the synergistic effect of LFE and JLS on LPS-stimulated RAW 264.7 cells. Our finding indicated that addition of JLS to LFE resulted in an augmentation of the anti-inflammatory properties of LFE. It attenuated inflammatory mediators such as NO, iNOS, and COX-2 by decreasing NF-κB and MAPK activation compared to LFE-only treatment. These results suggest the possible application of combination of JLS and LFE as a promising candidate for an anti-inflammatory functional ingredient.