Introduction
Materials and methods
Chemical reagents
Sample preparation
Cell culture
MTT assay
Measurement of nitric oxide (NO)
Measurement of phagocytic activity
Reverse transcription-polymerase chain reaction (RT-PCR)
Animals and experimental design
Statistical analysis
Results and Discussion
HSL induces macrophage activation in RAW264.7 cells
HSL depends on TLR4 to increase the secretion of the immunostimulatory factors in RAW264.7 cells
HSL depends on p38 and JNK to increase the secretion of the immunostimulatory factors in RAW264.7 cells
HSL increases immune enhancement-related markers in immunosuppressed mouse models
Introduction
It is known that the immune response plays an important role in preventing and treating diseases caused by foreign pathogens (Hong et al., 2017). Since immunostimulants can enhance the host’s defense response to improve resistance to diseases caused by external pathogens, interest in the search for active substances that stimulate immune responses is increasing (Yu et al., 2016).
Among the various immune cells, macrophages, which are innate immune cells, play an important role in the first defense against foreign pathogens (Geng et al., 2018). Activated macrophages directly remove invading foreign pathogens through phagocytosis (Geng et al., 2018). In addition, macrophages recognize foreign pathogens and provide antigen- specific information to T cells or B cells which are adaptive immune cells (Zhou et al., 2021). In addition, cytokines secreted by macrophages during immune response induce activation of adaptive immune cells such as T cells and B cells (Zhou et al., 2021).
Hibiscus syriacus L. (H. syriacus) belonging to the Malvaceae family is the national flower of Republic of Korea and a medicinal plant that has been used to treat various diseases such as tinea, eczema, scabies, and dysentery (Shi et al., 2014). H. syriacus has been reported to have various pharmacological activities such as anti-cancer activity, anti- inflammatory activity, antioxidant stress activity, and anti-brain disease (Karunarathne et al., 2020). Although the immune-enhancing activity of plants of the genus Hibiscus has been reported (Geum et al., 2021; Shen et al., 2017), there is no study on the immune-enhancing activity of H. syriacus. Thus, we evaluated the immunostimulatory activity of H. syriacus and investigated its mechanism of action in mouse macrophages, RAW264.7 cells, and immunosuppressed mice.
Materials and methods
Chemical reagents
Specific inhibitors such as SB203580 (p38 inhibitor), PD98059 (ERK1/2 inhibitor), SP600125 (JNK inhibitor),) C29 (TLR2 inhibitor) and TAK-242 (TLR4 inhibitor) were purchased from Sigma Aldrich (St. Louis, MO, USA). Neutral red for phagocytosis analysis of RAW264.7 macrophages and cyclophosphamide as immunosuppressant were purchased from Sigma Aldrich. Primary and secondary antibodies for Western blot analysis were purchased from Cell Signaling (Bervely, MA, USA).
Sample preparation
The flowers, buds, leaves, and branches from H. syriacus were provided from Forest Medicinal Resources Research Center, Korea. Twenty grams of the flowers, flower buds, leaves, and branches from H. syriacus were immersed in 400 ml of distilled water and extracted for 12 h at 40℃. After extraction, the extracts were centrifuged for 10 min at 15,000 rpm, and then the supernatant was taken and lyophilized. The water extracts of flowers (HSF, yield=16.4%), flower buds (HSFB, yield=15.9%), leaves (HSL, yield=17.5%), and branches (HSB) from H. syriacus were stored in a refrigerator (-80 ℃) until use. Each extract was dissolved in distilled water and treated to RAW264.7 cells and immunosuppressed mice.
Cell culture
RAW264.7 cells purchased from American Type Culture Collection (Manassas, VA, USA) were maintained using Dulbecco’s Modified Eagle medium (DMEM)/F-12 1:1 Modified medium (Lonza, Walkersville, MD, USA) containing 10% fetal bovine serum, 100 U/mL penicillin and 100 ㎍/mL streptomycin at 37℃ under a humidified atmosphere of 5% CO2.
MTT assay
To evaluate the cytotoxicity of HSL, an MTT assay was performed. Briefly, RAW264.7 cells (5×105 cells/mL) cultured for 24 h in a 96-well plate were treated with HSL, and then further cultured in an incubator (5% CO2, 37℃, Humidified atmosphere) for 24 h. After 24 h, 50 μl of MTT solution (1 ㎎/mL, Sigma-Aldrich, St. Louis, MO, USA) was added to each well and then incubated in an incubator (5% CO2, 37℃, Humidified atmosphere) for 4 h. After 4 h, the culture medium was removed, and then 100 μl of dimethyl sulfoxide was added to each well to dissolve the purple formazan. The absorbance of dissolved purple formazan was measured at 570 ㎚ using a UV/Visible spectrophotometer (Human Cop., Xma-3000PC, Seoul, Korea).
Measurement of nitric oxide (NO)
The effect of HSF, HSFB, HSL, or HSB on the production of NO in RAW264.7 cells was evaluated using the Griess assay. Briefly, RAW264.7 cells were treated with HSF, HSFB, HSL, or HSB for 24 h. After 24 h, the cell culture supernatants and Griess reagent (Sigma Aldrich) were mixed and reacted at room temperature for 15 min. After 15 min, the absorbance was measured at 540 ㎚ using a UV/Visible spectrophotometer (Human Cop., Xma-3000PC, Seoul, Korea).
Measurement of phagocytic activity
The effect of HSL on the phagocytic activity in RAW264.7 cells was evaluated using a neutral red uptake assay. Briefly, RAW264.7 cells were treated with HSL for 24 h. After 24 h, RAW264.7 cells were washed three times with 1 X PBS and then stained with 0.01% neutral red solution for 2 h. After 2 h, RAW264.7 cells were washed three times with 1 X PBS and then absorbed neutral red was eluted with a lysis buffer (50% ethanol acid: 1% acetic acid = 1:1). The absorbance was measured at 540 ㎚ using a UV/Visible spectrophotometer (Human Cop., Xma-3000PC, Seoul, Korea).
Reverse transcription-polymerase chain reaction (RT-PCR)
The effect of HSF, HSFB, HSL, or HSB on the expression of inducible nitric oxide synthase (iNOS), interleukin-1β (IL-1β), and tumor necrosis factor-α (TNF-α) in RAW264.7 cells was evaluated using RT-PCR. Briefly, RAW264.7 cells were treated with HSF, HSFB, HSL, or HSB for 24 h. After 24 h, RAW264.7 cells were washed three times with 1 X PBS. Then, cDNA was synthesized using a Verso cDNA Kit (Thermo Scientific, Pittsburgh, PA, USA) from 1 ㎍ of total RNA extracted from RAW264.7 cells using an RNeasy Mini Kit (Qiagen, Valencia, CA, USA). Then, PCR was performed using a PCR Master Mix Kit (Promega, Madison, WI, USA), and the sequences of the primers such as iNOS, IL-1β, TNF-α, and GAPDH were as Table 1. The density of mRNA bands was calculated using the software UN-SCAN-IT gel version 5.1 (Silk Scientific Inc. Orem, UT, USA).
Table 1.
The sequences of the primers used in this study
Animals and experimental design
All animal procedures were conducted in accordance with the guidelines for the care and use of laboratory animals issued by Andong National University. Six-week-old male BALB/c mice were purchased from Orient (Sungnam, Korea). All mice were housed at 25 ± 2℃ and 50 ± 10% humidity under a 12-h light/dark cycle. The mice were randomly assigned to 10 mice per group (Control group, Cyclophosphamide group, HSL 50 ㎎/kg group, HSL 100 ㎎/kg group, and HSL 200 ㎎/kg group). Cyclophosphamide (80 ㎎/kg mice) was intraperitoneally injected to mice daily for 3 days to suppress the immunity of mice. After 3 days, HSL was orally administered every day for 10 days. At the end of the experiment, mice were sacrificed, and then the spleens and cardiac blood from all mice were collected. The spleens were weighted immediately after sacrifice and the whole blood samples were placed in VacutainerTM tubes containing EDTA (BD science, San Jose, NJ, USA). The numbers of white blood cells, neutrophils, lymphocytes, and monocytes were analyzed using a BC-5000 Vet Auto hematology analyzer (Nanshan, Shenzhen, China) in accordance with the manufacturer’s recommendation. In addition, the levels of IL-1β, IL-6, and TNF-α from the serum of mice were analyzed by ELISA kit (Cayman Chemical, Ann Arbor, Michigan, USA) in accordance with manufacturer’s protocols.
Statistical analysis
All the data are shown as mean ± SD (standard deviation). Statistical significance was determined by Student’s t-test (EXCEL, Microsoft Corporation, Redmond, Washington, USA). Differences with *P < 0.05 or #P < 0.05 were considered statistically significant.
Results and Discussion
HSL induces macrophage activation in RAW264.7 cells
It is reported that macrophages involved in the innate immune response recognize invaded foreign pathogens and remove them through phagocytosis (Kasimu et al., 2017; Yu et al., 2021). When foreign pathogens invade, macrophages secrete various immunostimulatory factors such as NO, iNOS, IL-1β, and TNF-α to eliminate them (Ma et al., 2016; Siveen and Kuttan, 2009). It has been reported that NO produced by iNOS directly attacks foreign pathogens (Ren et al., 2017). In addition, IL-1β and TNF-α have been known to strengthen the adaptive immune response by activating T cells and B cells (Zha et al., 2018). Thus, it is reported that the activation of macrophages to induce secretion of these immunostimulatory factors contributes to host defense against external pathogens (Zhang et al., 2013).
In this study, we investigated the effect of H. syriacus on the secretion of immunostimulatory factors such as NO, iNOS, IL-1β, and TNF-α in RAW264.7 cells to confirm whether H. syriacus has an immune-enhancing activity. First, we compared the effect of flowers (HSF), flower buds (HSFB), leaves (HSL), and branches (HSB) of H. syriacus L. on the secretion of the immunostimulatory factors. As shown in Fig. 1A, HSL and HSB dramatically increased the production of NO, iNOS, IL-1β, and TNF-α in RAW264.7 cells. HSF has been shown to increase the production of iNOS and TNF-α in RAW264.7 cells. However, HSFB did not increase any of NO, iNOS, IL-1β, and TNF in RAW264.7 cells. From the results (Fig. 1A), we selected HSL for further study. HSL dose-dependently increased the production of NO, iNOS, IL-1β, and TNF-α in RAW264.7 cells (Fig. 1B).
Since macrophages remove foreign pathogens through phagocytosis, phagocytosis activation of macrophages is considered to mean immune enhancement (We et al., 2006). Thus, we investigated the effect of HSL on macrophage phagocytic activity in RAW264.7 cells. As shown in Fig. 1C, HSL dose-dependently increased the phagocytic activity in RAW264.7 cells. In addition, HSL did not affect the cytotoxicity of RAW264.7 cells (Fig. 1D). These results (Fig. 1A- 1C) suggest that HSL may activate macrophages which may contribute to the enhancement of innate immune responses.
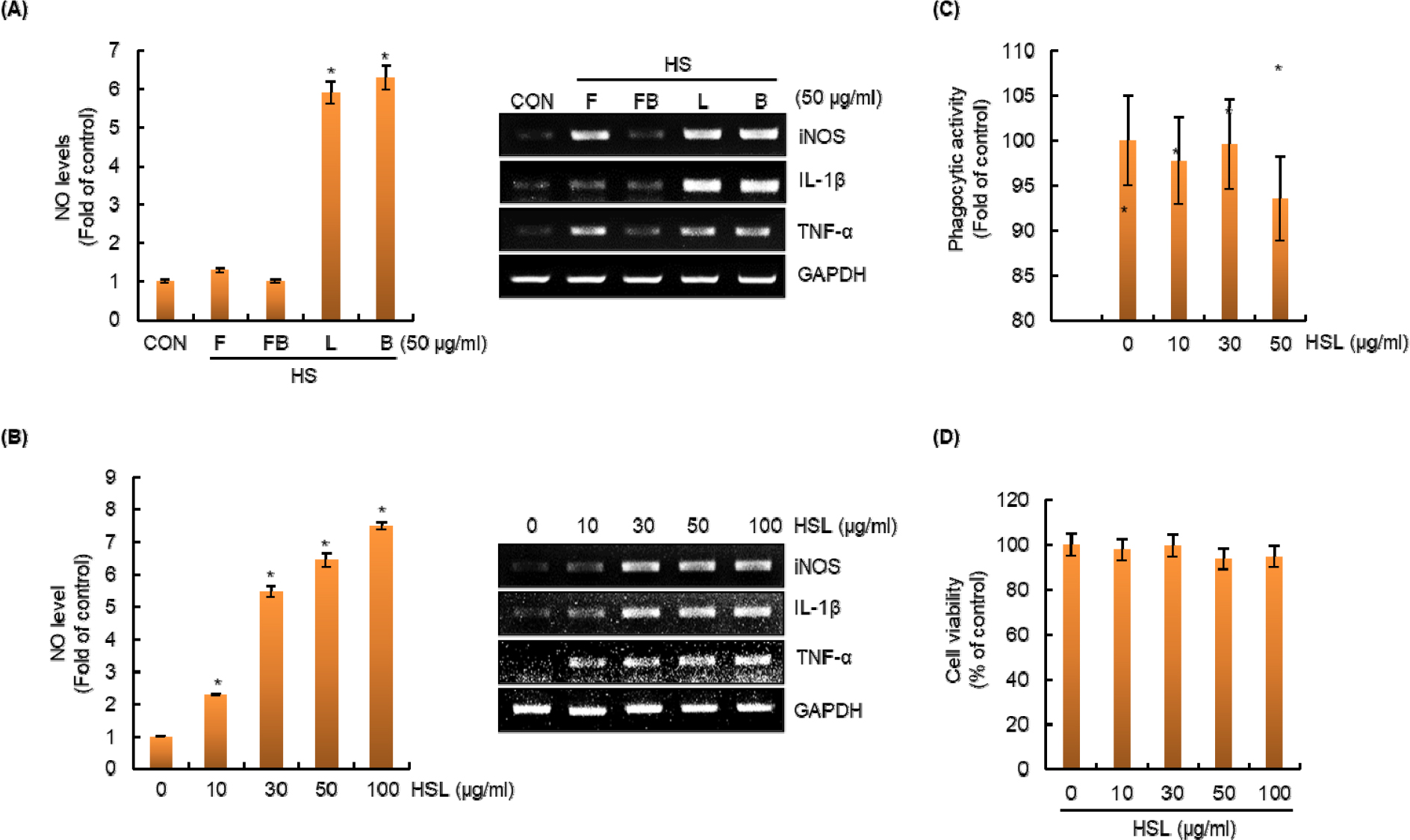
Fig. 1.
Effect of HSL on the macrophage activation in RAW264.7 cells. (A) After RAW264.7 cells were treated with HSF, HSFB, HSL or HSB for 24 h, the NO level and mRNA level were measured by Griess assay and RT-PCR, respectively. *P < 0.05 compared to the cells without the treatment. (B) After RAW264.7 cells were treated with HSL for 24 h, the NO level and mRNA level were measured by Griess assay and RT-PCR, respectively. *P < 0.05 compared to the cells without the treatment. (C) RAW264.7 cells were treated with HSL for 24 h. the absorbance of neutral red was investigated using a microplate reader. *P < 0.05 compared to the cells without the treatment. (D) RAW264.7 cells were treated with HSL for 24 h. The cytotoxicity of HSL to RAW264.7 cells was performed using MTT assay. *P < 0.05 compared to the cells without the treatment.
HSL depends on TLR4 to increase the secretion of the immunostimulatory factors in RAW264.7 cells
Toll-like receptors (TLRs) have been reported to play a key role in regulating macrophage activation (Swanson et al., 2020). TLRs recognize pathogen-associated molecular patterns (PAMPs) of foreign pathogens and induce an inflammatory response to efficiently eliminate foreign pathogens (Moynagh, 2005; Swanson et al., 2020). Thus, natural products that stimulate TLRs were searched for macrophage activation, and it was reported that various natural products induce macrophage activation through stimulation of TLR2 and TLR4 among TLRs (Bai et al., 2019; Shen et al., 2017; Yang et al., 2019; Zhang et al., 2013). Thus, we investigated whether TLR2 and TLR4 were involved in the production of the immunostimulatory factors by HSL in RAW264.7 cells. As shown in Fig. 2, NO produced by HSL was reduced in TLR2 inhibition by C29, but there was no effect on the production of iNOS, IL-1β, and TNF-α in RAW264.7 cells. However, inhibition of TLR4 by TAK-242 markedly reduced the production of NO, iNOS, IL-1β, and TNF-α.
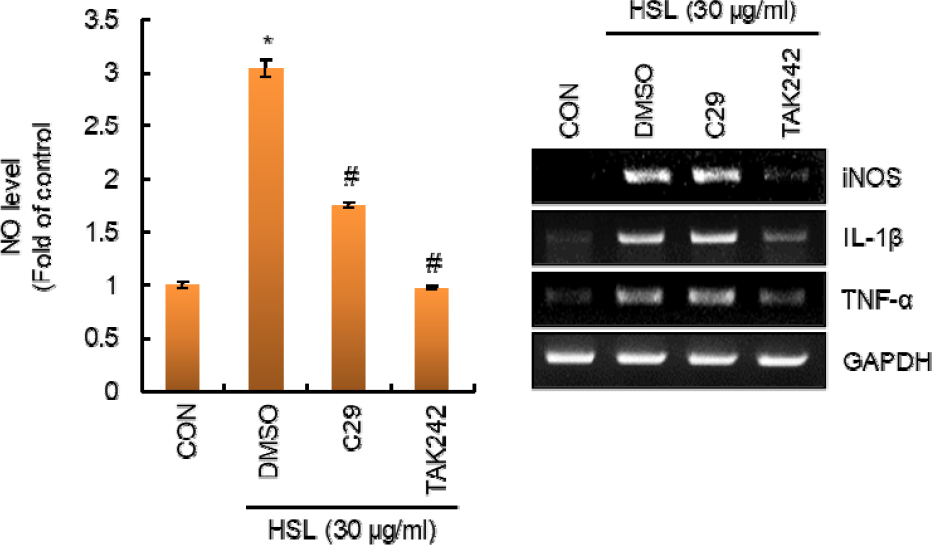
Fig. 2.
Effect of TLR2/4 on the HSL-induced production of immunostimulatory factors in RAW264.7 cells. RAW264.7 cells were pretreated with C29 (TLR2 inhibitor, 100 μM) or TAK-242 (TLR4 inhibitor, 5 μM) for 2 h and co-treated with HSL (30 ㎍/mL) for 24 h. The NO level and mRNA level were investigated using Griess assay and RT-PCR. *P < 0.05 compared to the cells without the treatment. #P < 0.05 compared to the cells with HSL alone.
From these results, it is thought that HSL may induce the activation of macrophages mainly through the stimulation of TLR4.
HSL depends on p38 and JNK to increase the secretion of the immunostimulatory factors in RAW264.7 cells
Mitogen-activated protein kinases (MAPKs), composed of p38, extracellular signal‑regulated protein kinase 1/2 (ERK1/2), and c-Jun N-terminal kinase (JNK), are known as one of the major signaling pathways involved in the secretion of immunostimulatory factors by macrophages (Shen et al., 2017). Moreover, it has been reported that TLRs induce the activation of macrophages by activating MAPK signaling (Shen et al., 2017). Thus, we investigated whether MAPKs were involved in the production of the immunostimulatory factors by HSL in RAW264.7 cells. As shown in Fig. 3, there was no change in the production of immunostimulatory factors such as NO, iNOS, IL-1β, and TNF-α in the inhibition of ERK1/2 by PD98059. However, inhibition of p38 by SB203580 decreased the production of NO and IL-1b by HSL, and inhibition of JNK by SP600125 decreased the production of NO, iNOS, IL-1β, and TNF-α by HSL.
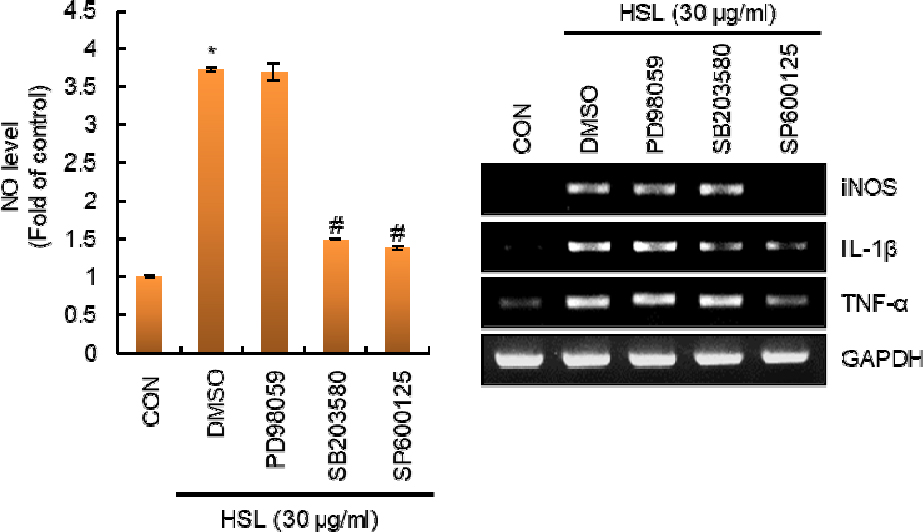
Fig. 3.
Effect of MAPK signaling pathway on the HSL-induced production of immunostimulatory factors in RAW264.7 cells. RAW264.7 cells were pretreated with PD98059 (ERK1/2 inhibitor, 40 μM), SB203580 (p38 inhibitor, 40 μM) or SP600125 (JNK inhibitor, 40 μM) for 2 h and then co-treated with HSL (30 ㎍/mL) for 24 h. NO level and mRNA level were measured by Griess assay and RT-PCR, respectively. *P < 0.05 compared to the cells without the treatment. #P < 0.05 compared to the cells with HSL alone.
From these results, it is thought that p38 and JNK are signaling pathways involved in the HSL-mediated activation of macrophages.
HSL increases immune enhancement-related markers in immunosuppressed mouse models
To verify the immune-enhancing activity of HSL in an animal model, immunosuppressed mice were treated with HSL, and then the immune enhancement-related markers were analyzed.
The spleen is an organ in the body that plays an important role in the innate and acquired immune systems (Aliyu et al., 2021). It is known that various immune cells such as B cells, natural killer cells, and macrophages exist in the spleen (Aliyu et al., 2021). Therefore, the increase in the spleen index in the immunosuppressed mouse model has been utilized as an immune enhancement index. In this study, we found that treatment of cyclophosphamide (CP) as an immunosuppressant reduced the splenic index, but HSL restored the reduced splenic index by CP (Fig. 4A).
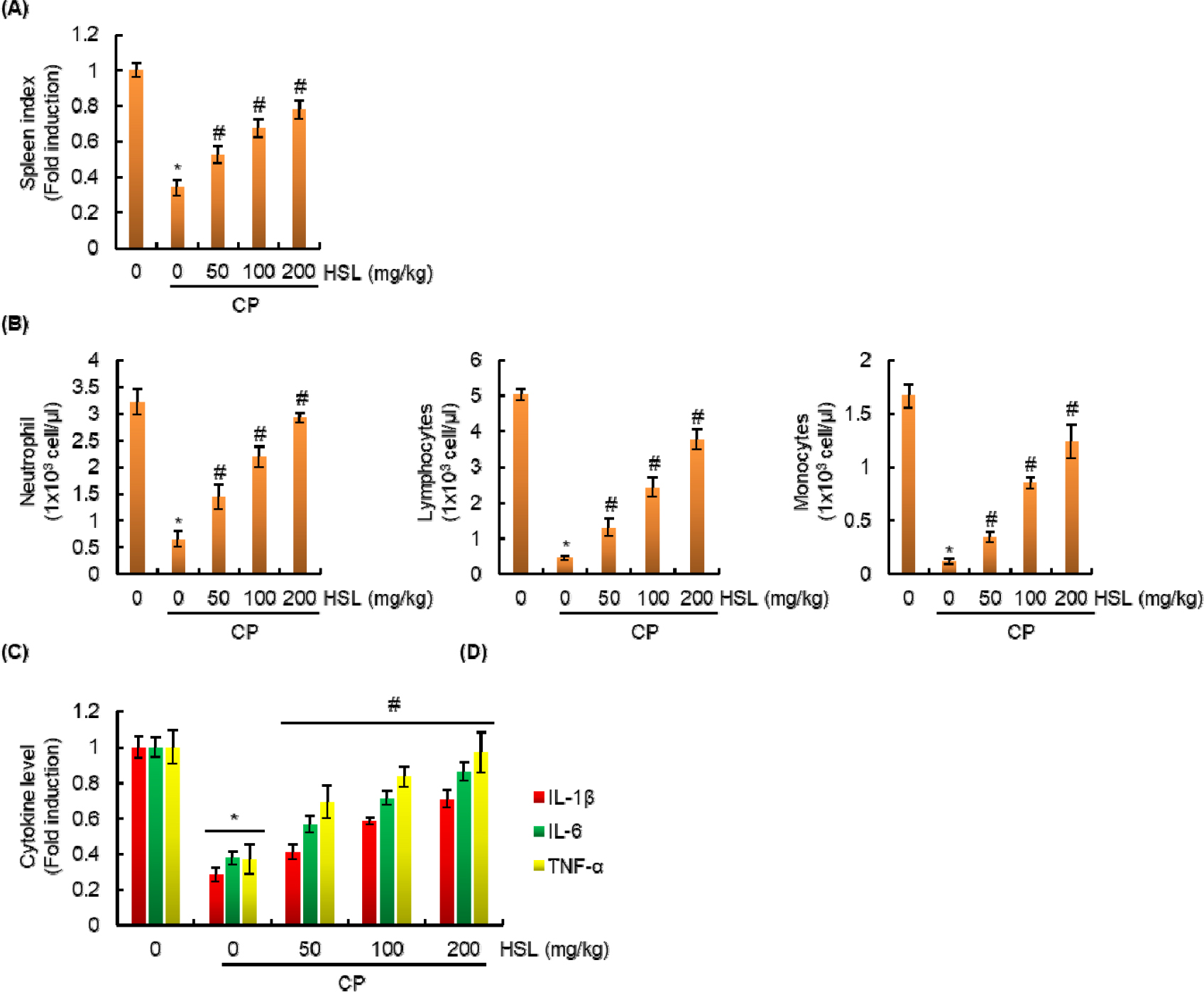
Fig. 4.
Effect of HSL on the immune-enhancement-related markers in immunosuppressed mice. (A) The spleen index was calculated as spleen wight/body weight. (B) The numbers of lymphocytes, neutrophils, and monocytes were analyzed using a BC-5000 Vet Auto hematology analyzer. (C) The levels of IL-1β, IL-6 and TNF-α were measured from the serum of the blood using ELISA kits. *P < 0.05 compared to the cells without the treatment. #P < 0.05 compared to the cells with HSL alone.
Blood is called the pipeline of the immune system because it contains various types of immune cells such as lymphocytes, neutrophils, and monocytes and immunostimulatory factors such as IL-1β, IL-6, and TNF-α (Geum et al., 2021). Thus, we analyzed the number of lymphocytes, neutrophils, and monocytes in the blood. As a result (Fig. 4B), treatment with CP alone decreased the number of lymphocytes, neutrophils, and monocytes compared to the normal group, but administration of HSL restored the reduced immune cells by CP such as lymphocytes, neutrophils, and monocytes. In addition, the administration of HSL increased the levels of IL-1β, IL-6, and TNF-α in the serum of the blood.
In summary, HSL increased the production of NO, iNOS, IL-1β, and TNF-α through the TLR4-dependent activation of p38 and JNK in RAW264.7 cells. In addition, HSL was shown to increase the spleen index, the numbers of lymphocytes, neutrophils, and monocytes, and the levels of the cytokines (IL-1β, IL-6 and TNF-α) in the immunosuppressed mouse model. These findings indicate that HSL may have immunostimulatory activity. Therefore, HSL may be considered to have immune-enhancing activity, and HSL is expected to be used as a potential immune-enhancing agent.